Emerging concepts in vaccine adjuvants
- Normal Liver Cells Found to Promote Cancer Metastasis to the Liver
- Nearly 80% Complete Remission: Breakthrough in ADC Anti-Tumor Treatment
- Vaccination Against Common Diseases May Prevent Dementia!
- New Alzheimer’s Disease (AD) Diagnosis and Staging Criteria
- Breakthrough in Alzheimer’s Disease: New Nasal Spray Halts Cognitive Decline by Targeting Toxic Protein
- Can the Tap Water at the Paris Olympics be Drunk Directly?
Emerging concepts in vaccine adjuvants
Emerging concepts in vaccine adjuvants. Adjuvants are important components of vaccines that enhance the degree, breadth and durability of immune responses.
After its introduction in the 1920s, alum remained the only adjuvant approved for human use for the next 70 years. Since the 1990s, five other adjuvants have been included in the licensed vaccines, but the molecular mechanisms by which these adjuvants work are still only partially understood.
However, our understanding of the activation of the innate immune system through pattern recognition receptors (PRR) is improving our understanding of adjuvant immune mechanisms, and recent conceptual advances have highlighted the concepts of tissue damage, different forms of cell death, and metabolism. Nutrition sensors can also regulate the innate immune system to activate adaptive immunity.
In addition, the latest advances in the use of systems biology to explore the molecular networks that drive the immune response of vaccines have also revealed relevant mechanisms and provided new ideas for the discovery and development of vaccines.
This article reviewed the “now” and “future” of adjuvants, discussed some emerging concepts, and emphasized how our expanding knowledge of innate immunity and systemic vaccinology can be actively used in the development of new adjuvants for COVID-19 vaccines Scientific development and development of adjuvants for future epidemic vaccines.
Generalize
An adjuvant is a substance added to a vaccine to stimulate and enhance the strength and durability of the immune response.
Vaccines generally include two components: antigen and adjuvant. Antigens are usually composed of proteins or compounds derived from pathogens to which the body needs to produce an adaptive immune response. An adjuvant is a substance added to a vaccine to stimulate and enhance the strength and durability of the immune response.
The word adjuvant (derived from the Latin “adjuvare”, meaning “help”) was coined by French veterinarian Gaston Ramon, who discovered it while working at the Pasteur Institute in 1920. Surprisingly, vaccines developed using aluminum salts produced better antibody responses than soluble toxoids. Since then, aluminum adjuvants have been used in billions of vaccines, and millions of people are vaccinated each year.
The development of new vaccine adjuvants has been described as one of the slowest processes in medical history. In the more than seven years since the initial license in the 1920s, insoluble aluminum salts (alum) have remained the only adjuvant contained in vaccine products, such as those for hepatitis B, diphtheria, tetanus and whooping cough or human papilloma virus. vaccine. However, in the late 1990s, the oil-in-water emulsion adjuvant MF59 first appeared in a European licensed product called Fluad, which is a trivalent inactivated vaccine against seasonal influenza and can be used for people over 65 years of age. Adults.
In the following 20 years, four other adjuvants have been included in other vaccine products: AS01 (for shingles vaccine Shingrix and malaria vaccine Mosquirix), AS04 (for hepatitis B vaccine Fendrix and human papilloma vaccine Cervarix) ), AS03 (for pandemic influenza vaccines Pandemrix and Arepanrix) and cytosine guanosine phosphate (CpG) 1018 (for hepatitis B vaccine Heplisav-B). Although many other adjuvants have shown high potency in preclinical models during this period, most of them have not yet been licensed, mainly due to safety or tolerability considerations. In addition, although they are widely used, the molecular mechanisms by which available adjuvants (including alum, MF59 and AdjuvantSystems AS0 adjuvant) actually work in the human body are unclear.
Nevertheless, the past two decades have witnessed a revolution in how our innate immune system infects microorganisms, which provides a huge opportunity for further understanding of adjuvant design and development. In the late 1990s, it was discovered that the activation of receptors such as Toll-like receptors (TLR), dendritic cells (DC) and highly conserved pathogen-associated molecular patterns (PAMP) in microorganisms caused DC activation and stimulated antigen specificity. T and B cell response. These findings provide strong experimental evidence for the concept proposed by Charlie Janeway in 1989: the innate immune system perceives microorganisms through recognition receptors (PRR; such as TLR).
These receptors recognize PAMP and lead to the activation of innate immune cells and subsequent adaptation. immune response. In the ten years after the discovery of TLR, other PRRs (such as retinoic acid-inducible gene I (RIG-I) and other RNA sensors), DNA sensors (such as interferon gene stimulator (STING) protein), C-type lectins, Nucleotides bind to oligomerization domain (NOD)-like receptors (NLR) and cytoplasmic receptors, such as NLRP3, which activate inflammasomes and have also been found and shown to affect adaptive immunity. Molecules that target these receptors are also considered as potential vaccine adjuvants.
Despite these major advances, our understanding of how the available adjuvants contained in licensed vaccines actually work is still imperfect. However, two major developments in the past decade have begun to develop a deeper understanding of the mechanism of adjuvants. First of all, immunologists’ views on how to stimulate the immune system have gone beyond the PAMP pattern recognition model originally proposed by Janeway. Therefore, there is evidence that DC and other cells of the innate immune system can not only be activated by pathogens through PRR, but also through tissue damage that causes the release of damage-related molecular patterns (DAMP), through cell death, and other stress signals, such as Amino acid starvation (through paleo-stress and nutrient sensory pathways).
Many of these stimuli have been shown to induce adaptive immunity, thus providing support for the “danger model” concept proposed by PollyMatzinger in 1994. Second, using systems biology methods to detect the innate and adaptive immune response to vaccines, and to define the molecular network that drives the innate and adaptive immune response to vaccination in humans, and begin to generate basic ideas on how to initiate and control the immune response. Insights into new mechanisms. This “systematic vaccinology” method is now increasingly used to assess human immune response to vaccination, to determine the molecular features that can predict vaccine efficacy and gain insights into the mechanism.
In this review, we discuss how these advances study adjuvant science. First, we discussed the known experience and gaps in adjuvants in licensed products. Second, we discussed several emerging concepts regarding the immunological mechanism of adjuvant action. In the last section, we provide a conceptual framework in which we emphasize how a system-based approach can assist in the design and development of adjuvants.
Licensed vaccine adjuvant
Endogenous adjuvants in live vaccines
Although there are only a handful of adjuvants available for clinical use, many vaccines that have been safely used in billions of people contain endogenous adjuvants. The advancement in the concept of innate immunity and the research on the key innate receptor PRR have made people realize that many widely used live vaccines induce immune responses by activating specific PRRs. These vaccines are composed of live attenuated pathogens that activate innate immunity by expressing various PAMPs. For example, Yellow Fever Live Attenuated Vaccine (YF-17D) is one of the most effective vaccines in history. It has been developed and used in 600 million people worldwide, and has been proven to pass multiple TLRs (TLR2, TLR3 and TLR7) Send a signal to activate the innate immune system.
TLR9 and through RIG-I and melanoma differentiation-associated protein 5 (MDA5) ( Figure 1 below ). Importantly, TLR signaling is also necessary for the immunogenicity of YF-17D, although the additional impact of sustained antigen expression through attenuated viral replication should not be underestimated. Similarly, Bacille Calmette-Guerin (BCG) prevents tuberculosis, where more than four billion doses have been used, to activate TLR2, TLR4, TLR9 and CD209 antigens (also known as DC-SIGN). In addition, certain inactivated vaccines can also trigger TLRs, such as seasonal influenza vaccines that activate the innate immune system through the TLR7 and myelodifferentiation primary response 88 (MyD88) signaling pathway.
These findings further indicate the continued rational development of TLR agonists as key targets in adjuvant design, especially in the context of subunit vaccines without endogenous adjuvants. Many studies have demonstrated the efficacy of synthetic TLR ligands as vaccine adjuvants in mice and non-human primates (NHPs), which can promote the enhancement and improvement of vaccination against recombinant protein antigens, virus-like particles or DNA-encoded antigens. Long-lasting antibody response.
Figure 1: Molecular targets of adjuvants. Figure a, Toll-like receptors (TLR) TLR1, TLR2, TLR4, TLR5 and TLR6 are expressed on the cell surface, while TLR3, TLR7, TLR8 and TLR9 are expressed in endosomes. TLR1 and TLR6 heterodimerize with TLR2, and send signals through the Myeloid Differentiation Primary Response 88 (MyD88) pathway to activate NF-κB and MAP kinases, leading to the secretion of pro-inflammatory and anti-inflammatory cytokines. TLR4 and TLR5 function as homodimers and send signals through the MyD88 pathway. TLR7 and TLR9 also use the MyD88 pathway, but will quickly activate IRF7 to induce type I interferon. TLR3 uses a linker containing the TIR domain to induce IFNβ (TRIF) signals through IRF3 to induce type I interferons. Figure b.
The cytosolic pattern recognition receptor (PRR) is a sensor of pathogen-related molecular patterns (PAMP) and damage-related molecular patterns (DAMP) that exist in the cytoplasm. Nucleotide-binding oligomerization domain (NOD)-like receptor (NLR) is a cytoplasmic sensor of bacterial PAMP, but it also recognizes a variety of cell products, including ATP, uric acid, and K+ to activate the NF-κB pathway and induce driving T Helper cell 2 (TH2) cell differentiation. Retinoic acid inducible gene I (RIG-I) and melanoma differentiation-associated protein 5 (MDA5) are intracellular viral sensors that drive type I interferon response through IRF3 and IRF7. The cGAS interferon gene stimulator (STING) pathway recognizes double-stranded DNA (dsDNA) to induce the NF-κB pathway.
Figure c. C-type lectin receptor (CLR) is a cell surface molecule expressed on multiple bone marrow cell subpopulations. Dectins1 and 2 and MINCLE recruit SYK1 and activate NF-κB through the CARD9-BCL-10-MALT1 complex. In addition, dectin has been shown 1 Induce NFAT and AP1 pathways in macrophages and dendritic cells (DC) and in vitro experimental models, respectively. Dectins are also specifically used to induce anti-fungal immunity. Dendritic cell-specific ICAM3 grabbing non-integrin 1 (DC-SIGN) activates NF-κB through p65 acetylation; however, although it has been shown to induce IL-10 expression, little is known about the resulting gene expression . It is known that DEC205 and DNGR1 can induce cross-presentation, but the signaling pathway is unknown. ASC, apoptosis-related spot-like protein containing CARD; CpG, cytosine guanosine phosphate; double-stranded RNA, double-stranded RNA; LPS, lipopolysaccharide; ssRNA, single-stranded RNA; TDM, trehalose-6,6-dimycete Acid ester; TReg cell, regulatory T cell.
Live virus vaccines usually induce a strong and long-lasting antibody response in humans, and it is possible that adjuvants with inactivated vaccines can induce such a strong and long-lasting antibody response. Based on the discovery that the yellow fever vaccine binds to multiple DC subgroups through multiple TLR ligands, we designed synthetic nanoparticles containing TLR4 and TLR7/TLR8 ligands as adjuvants, and soluble protein antigens (such as chicken eggs). Albumin) can be used in combination or flu hemagglutinin can also be used. Experiments in mice have shown that these nanoparticle vaccines induce a strong and long-lasting antigen-specific antibody response: germinal center (GC) response, T follicular helper (TFH) cell response and long-lived plasma cell (LLPC).
It is worth noting that these strong reactions caused by the combination of TLR ligands lasted a lifetime in mice, and required the activation of multiple DC subsets and direct activation of B cells. Subsequent studies in the NHP showed that such nanoparticle-encapsulated TLR ligands are effective adjuvants for stimulating robust and durable antibody responses, GC and TFH cellular responses, and continued until the study was terminated at the high frequency of LLPCs at 70 weeks . It seems that the production of LLPCs may be the key to the durability of the antibody response, and adjuvants that drive the differentiation of LLPCs will be of particular interest in vaccinology. Therefore, the development of adjuvants to promote LLPCs is a major challenge in vaccinology.
Recent work has shown that sustained-release delivery systems (such as osmotic pumps) that release antigens with delayed kinetics can enhance the magnitude, quality, and durability of antibody responses. Although this is due to the enhanced GC response due to the longer retention time of the antigen in the lymph nodes, the effect of adjuvants on the continuous activation of innate cells has not been thoroughly evaluated. In this case, it was discovered that 3M-052, a new type of TLR7/TLR8 agonist slowly released from the site of administration, leads to the activation of monocytes and DC for 3-4 weeks, and induces a sustained antibody response and LLPCs ratio. The observation with alum was much higher (up to 100 times), and lasted until the end of the study 70 weeks after immunization.
Alum
Alum is the most commonly used adjuvant to induce antibody responses and CD4+ human T helper cell responses ( Table 1 below ). These T helper responses are T helper 2 (T ħ 2) cells biased in mice, but this deviation is not clear in humans. For a long time, it has been believed that alum mainly mediates its adjuvant effect through a “storage effect” mechanism, which involves the slow release of antigens from immune sites. Consistent with this, compared with traditional alum adsorption of antigens, chemical modification of antigens with short peptides composed of repeated phosphoserine (pSer) residues can enhance the binding to alum, thereby prolonging the bioavailability of the immunogen, and greatly Enhance GC and antibody response.
In addition, alum actually has multiple effects on the immune system. As mentioned above, although TLR seems to be critical to the immunogenicity of live virus vaccines such as yellow fever vaccine YF-17D, the immune response of alum plus antigen immunostimulation is in mice lacking the key adaptor protein involved in TLR signaling. Unaffected: MyD88 or a linker containing the TIR domain induces IFNβ (TRIF). This indicates that the adjuvant effect of alum occurs through a mechanism independent of TLR signaling. Subsequent studies have shown that alum activates the NLRP3 inflammasome, although data on the relative importance of this in mediating the adjuvant effect of alum are conflicting. Evaluation of alum enhanced immune response in mice deficient in signaling NLRP3 experiment seems to give NLRP3 inflammasome activation whether the adaptive immune response is necessary to conflicting results (see Table 1 ).
Table 1. “Known” and “Unknown” of adjuvants used in licensed vaccines.
Adjuvant name | Known mechanism | Unknown mechanism |
Alum (aluminum hydroxide, aluminum phosphate) | Antibody responds to the activation of macrophage NLRP3 inflammasome in independent TLR signals; and the activation of DC is the rapid recruitment of granulocytes mediated by uric acid and the formation of induced cell death to release DNA, which triggers STING-IRF3 activation, IgE antibody Required to react with TH2 cells. | The innate receptors and signal transduction leading to antibody and T helper cell responses are poorly understood, and the correlation between stress response signals, tissue damage, and metabolic and nutrient sensing pathways is poorly understood. The role of NLRP3 inflammasome in mediating adjuvant activity is poorly understood. There is conflicting evidence for the role. |
MF59 Squalene, Tween (Polysorbate) 80, Span 85 | Activating macrophages and DCs at the injection site induce chemokine secretion antibodies and CD4+ T cell responses depending on the transient release of ATP from muscle cells. MyD88 activation independent of TLR and ASC activation independent of NLRP3 stimulate antigen-specific CD8+ T cells in the tissue pass through the RIPK3-dependent pathway. | Little is known about the innate receptors and signals that lead to antibody and T helper cell responses, and little is known about the relevance of stress response signals, tissue damage, and metabolic and nutrient sensing pathways. |
AS04 (Alum+MPL) | Compared with alum, AS04-activated DCs enhance antigen presentation and MPL is essential for the activation of TLR4, and alum can prolong the response induced by TLR4. | Little is known about the innate receptors and signaling of alum that cause antibody and T helper cell responses |
AS03 squalene and α-tocopherol + Tween (polysorbate) 80 | It induces NF-κB activity and chemokine response in the local and draining lymph nodes of mice between 6 to 48 hours, and α-tocopherol activates human monocytes and macrophages. | Little is known about the innate receptors and signals that cause antibody and T helper cell responses, and the relevance of stress response signals to tissue damage and metabolic and nutrient sensing pathways is poorly understood. |
AS01 (MPL, QS-21 liposome) | NK cells and CD8+ T cells locally secrete the chemokine IFNγ in the draining LN, QS-21 activates caspase 1 in SSM, and induces monocytes to differentiate into heterogeneous DC populations responsible for the activation of T cells in draining LN . | Little is known about the innate receptors and signals that sense QS-21 and cause antibody and T helper cell responses, and little is known about the relevance of stress response signals, tissue damage, and metabolic and nutrient sensing pathways. |
CpG1018 (single-stranded DNA) | Activation of TLR9 leads to MyD88 pathway and type I interferon response. | too much |
Alum can also enhance adaptive immunity by causing tissue damage and inducing uric acid-mediated inflammatory DC activation. The injection of alum quickly caused various cells, including neutrophils, neutrophils, and extracellular traps composed of their chromatin. The release of DNA partly mediates the adjuvant activity of alum. For example, in mice, immunization with antigen plus alum can induce cell death and subsequent release of host cell DNA, thereby stimulating antigen-specific IgE responses and TH2 cell responses.
The TH2 cell response is related to IgE isotype switching through a mechanism that relies on TANK-binding kinase 1 (TBK1) and the transcription factor IRF3, which are key components of the signaling pathway mediated by the cytoplasmic DNA sensor STING ( Table 1 above ). In some of these studies, co-injection of DNase preparations with alum and antigen reduced the immune response to vaccination. However, a subsequent study reported that commercial DNase preparations were contaminated with proteases, which was responsible for some inhibitory effects of DNase preparations on the activity of alum adjuvants.
Nevertheless, DNase reduced the alum-induced response to a certain extent, but the effect of DNase seems to be independent of its ability to cut DNA. Therefore, future work should aim to clarify the relative importance of DNA sensing in mediating the induction of antibody and CD4+T helper cell responses, which are induced by alum plus antigen immunity. Alum is also used in inactivated virus COVID-19 vaccines that have been approved for limited or emergency use in certain countries ( Table 2 below ). Therefore, the mechanism of action of alum is complicated. In small animals, several factors may work at the same time, and the role of the preparation cannot be ignored.
Table 2. Vaccine adjuvants restricted for use in the COVID-19 vaccine or in clinical trials
Adjuvant | vaccine | manufacturer | How to use |
alum | Inactivated SARS-CoV-2 vaccine | Sinopharm Kexing | Approved for limited or emergency use in certain countries |
Matrix-M | Recombinant SARS-CoV-2S protein vaccine | Novavax | Phase III |
AS03 | Recombinant SARS-CoV-2 S protein as soluble protein or virus-like particles | GlaxoSmithKline (AS03) Sanofi (antigen) Medicago (antigen) | I/II III |
CpG 1018 | Virus-like particles recombinant SARS-CoV-2 S protein | Dynavax(CpG 1018)Medicago(抗原) | Phase I / II |
TLR7/TLR8 ligand adsorbed in alum | Inactivated SARS-CoV-2 vaccine | Bharath Biotech | Phase III (India)/emergency use |
MF59
MF59 is an oil-in-water emulsion adjuvant that has been included in licensed influenza vaccines in Europe since 1997 and is now used in more than 100 million people in more than 30 countries. This adjuvant contains squalene oil droplets, a biodegradable and biocompatible oil, which is a normal component of the human body. It is stabilized in an aqueous buffer by the surfactants Tween 80 and Span 85. They are commonly used Of non-ionic surfactants. Its mechanism of action has been explored and reviewed.
In short, the use of MF59 in muscle activates bone marrow cells (such as macrophages and DC), which produce chemokines such as CC-chemokine ligand 2 (CCL2), CCL4, CCL5, and CXC-chemokines. Chemokine ligand 8 (CXCL8, also known as IL-8), then recruits neutrophils, eosinophils, and more monocytes and DCs to the injection site (see Table 1 above ). These cells further amplify the response and migrate to draining lymph nodes to activate B and T cells. Studies in mice have shown that MF59 induces a wider range of cytokines and chemokines than alum, and rapidly recruits CD11b+ inflammatory cells to the injection site.
These observations based on small animal studies are largely confirmed by similar studies conducted in the NHP. The MF59 adjuvant influenza vaccine was injected into mice intramuscularly, resulting in a transient extracellular release of ATP. Local injection of adenosine triphosphate (a type of ATP hydrolase) inhibited cell recruitment and antigen-specific CD4+ T cell response. The antibody response was induced but not by alum or incomplete Freund’s adjuvant. These data indicate that the transient release of ATP induced by the MF59 adjuvant vaccine mediates the adaptive immune response.
Regarding the innate immune receptor involved in the perception of MF59, it is proved that the antibody response of MF59 adjuvant depends on MyD88, but is independent of NLRP3 inflammasome activation. The immune MYD88 knockout is used against a MF59 adjuvant vaccine against mouse Neisseria meningitis. Cocci (rMenB) resulted in lower antibody titers compared to those obtained in control mice. However, surprisingly, MF59 did not activate TLR signaling in the HEK293 cell line expressing TLRs, which led to the conclusion that MF59 induces antibody responses through a MyD88-dependent mechanism independent of TLRs.
In an independent study, the H5N1 subunit vaccine containing MF59 adjuvant was immunized into mice with a gene defect of apoptosis-related spot-like protein, which contains CARD (ASC), one of the NLRP3 inflammasomes. This adaptor protein leads to a reduction in H5-specific IgG antibody titers relative to the results observed in wild-type mice. Interestingly, this study is consistent, the response of Nlrp3-knockout and Casp1-knockout mice is complete, and the MF59 adjuvant vaccine induces an antibody response through an ASC-dependent but not inflammasome-independent pathway.
Finally, our recent work has shown that subcutaneous immunization of mice with MF59 plus antigen stimulates the antigen-specific CD8+ T cell response (in the draining lymph nodes it is stimulated at a very low amplitude, but can be stimulated at a higher level in the tissues). Frequency detection, such as lung and liver), is a key mediator of necroptosis through the mechanism of serine/threonine protein kinase 3 (RIPK3) that relies on receptor interaction. Therefore, immunizing mice with an antigen mixed with MF59 or its mimic Addavax, but not mixed with alum, induces rapid RIPK3-dependent necroptosis of lymph node macrophages. RIPK3-deficient mice have impaired ability to produce antigen-specific CD8+ T cell responses in the lung and liver in response to subcutaneous immunization with antigen plus Addavax.
However, surprisingly, this response is normal in mice lacking mixed lineage kinase domain-like protein (MLKL), which is a downstream mediator of necroptosis, which suggests that in RIPK3-deficient mice The impaired CD8+ T-cell response observed in is caused by an independent mechanism. In contrast to the effects observed on CD8+ T cells, the antibody response was not affected in RIPK3-deficient mice or caspase 1-deficient mice. However, they are affected by the pan-caspase inhibitor Z-VAD-FMK, which suggests that apoptotic caspase has an effect on the induction of Contribution of antibody response. These observations in mice provide insights into the mechanism of the adjuvant effect of squalene-based adjuvants (such as MF59), but the extent of this mechanism in humans remains to be determined.
AS0 adjuvant system
The adjuvant system AS0 was developed by GlaxoSmithKline in the past thirty years. It is based on a reasonable combination of classic adjuvant molecules (including alum, emulsions and liposomes) and used in combination with immunostimulatory molecules (such as TLR ligands, etc.).
AS04
AS04 is composed of 3-O-desacyl-4′-monophosphoryl lipid A (MPL), which is a form of lipopolysaccharide (LPS) extracted from Salmonella Minnesota, adsorbed on aluminum salt. Importantly, studies in mice have shown that when adsorbed on alum, MPL maintains its complete immunostimulatory activity through TLR4 activation. The adjuvant effect of AS04 is mediated by the signal transduction of TLR4 to innate cells and the innate immune modulatory properties of alum (see Table 1 above ).
Compared with the same antigen with only alum adjuvant, the addition of AS04 to the recombinant antigen-based hepatitis B virus (HBV) and human papillomavirus (HPV) vaccines can induce higher levels of antibodies, proving that the TLR4 agonist MPL Added value in humans. The higher immunogenicity also translates into the high and long-lasting efficacy of the HPV-16/18 vaccine. Now available data show that more than 9 years after the initial immunization, the effectiveness of the vaccine method can reach 100%. Further research is needed to better understand the exact mechanism behind this beneficial effect of the AS04 adjuvant HPV vaccine. For example, the addition of AS04 can lead to a broader immune response against different human HPV strains through a mechanism that has not been clearly defined.
AS03
AS03 is a squalene oil-in-water emulsion adjuvant, similar to MF59, but also contains α-tocopherol (vitamin E) as an additional immune-enhancing ingredient. AS03 and pandemic influenza vaccine are jointly licensed (see Table 1 above ). AS03 is evaluated in clinical trials as a vaccine adjuvant for many different recombinant viral spike protein COVID-19 vaccines (see Table 2 above ). AS03 enhances the range and breadth of antibody response and CD4+ T cell response, and enhances the protection against influenza compared with non-adjuvant vaccines. Studies in mice and humans have shown that AS03 triggers a short-lived innate immune response, although other mechanisms of action may also be involved.
In mice, AS0 stimulates the transient production of cytokines at the injection site and draining lymph nodes. Alpha-tocopherol regulates the expression of certain chemokines and cytokines, such as CCL2, CCL3, IL-6, granulocyte colony stimulating factor (G-CSF, encoded by CSF3) and CXCL1, and enhances antigen uptake by monocytes and Increase granulocytes to drain lymph nodes. A subsequent study in mice showed that intramuscular injection of AS03 caused rapid and transient down-regulation of genes involved in lipid metabolism in draining lymph nodes. In vitro, in bone marrow cell lines, these changes are related to changes in lipid composition, changes in endoplasmic reticulum (ER) morphology, and activation of the unfolded protein response (UPR) pathway.
In vivo, treatment with a chemical inhibitor of the endoplasmic reticulum stress response or gene deletion in the myeloid cells of the endoplasmic reticulum stress sensor kinase IRE1α can reduce IL-6 production. In humans, the AS03 adjuvant is administered Within 24 hours after the H5N1 avian influenza vaccine, the serum levels of IL-6 and IP10 (also known as CXCL10) as well as the transcriptional characteristics of interferon signaling and antigen processing and presentation in DC, monocytes and neutrophils increased.
Interestingly, it was found that this early up-regulation of the interferon signal can predict antibody titers at 56 days and 90 hours. Given that AS03 and MF59 are both squalene-based adjuvants, they are likely to participate in the common pathway of innate immune activation. As mentioned above, both of these adjuvants stimulate innate cytokines and chemokines, but the extent to which they both rely on IRE1α and RIPK3-dependent pathways remains to be determined.
AS01
AS01 is included in the licensed varicella-zoster vaccine (Shingrix), which is approved for use in the elderly (50 years and older) and is highly effective (97.2%). The adjuvant AS01 is a unique combination of two different immunostimulatory components-the TLR4 ligand used in AS04 (MPL) and the purified saponin fraction (QS-21)-also used in the licensed malaria vaccine, the vaccine It is currently being implemented in Africa with limited conditions. QS-21 is a triterpene glycoside purified from the bark extract of the Quillaja saponaria Molina tree. Preclinical studies using QS-21 adjuvant showed enhanced antibodies and cell-mediated immune responses. However, although QS-21 alone is effective, when used as a single-component adjuvant in human vaccines, there are major concerns about its tolerability.
In AS01, MPL and QS-21 are formulated together in liposomes in the presence of cholesterol. Cholesterol is used to bind QS-21 into liposomes and inhibit its reactogenicity. MPL activates the innate immune system through TLR4, mainly through TRIF-dependent signal transduction. In addition, studies on mice have shown that QS-21 activates caspase 1 in subcystic sinus macrophages (SSM) in draining lymph nodes 98 (see Table 1 above ).). Although the activation of caspase 1 by QS-21 is NLRP3-dependent in vitro, NLRP3 appears to have no effect in adjuvants in vivo. When formulated in liposomes, QS-21 enters cells through cholesterol-dependent endocytosis and induces lysosomal instability, followed by activation of tyrosine protein kinase SYK.
In general, the combination of the specific activation of different innate pathways by two separate molecules is essential for the complete adjuvant effect of AS01, because the consumption of TLR4, caspase 1, or SSM individually impairs the mouse model The adjuvant effect. Therefore, a significant feature of AS01 is that it produces a synergistic effect to induce new pathways, and these pathways are not triggered by any component alone. The key emergency pathway seems to be related to IFNγ, because blocking IFNγ in the body eliminates the synergistic effect of MPL and QS-21.
The typical immune response to AS01 adjuvant is characterized by the addition of multifunctional CD4+ T cells specific for the co-administered antigen (such as T cells expressing IL-2, IFNγ and TNF), and enhanced functional antibodies. In short, in AS01, two mature adjuvant molecules are combined in a new type of delivery system (liposomes), leading to the synergistic participation of innate immunity, which induces an adaptive immune response greater than the sum of individual components. Despite this, although a lot has been learned in preclinical models, there is still a lot to learn about how AS01 works in humans, especially in elderly subjects, in these licensed adjuvants , It seems to have the extraordinary ability to overcome “immune aging”.
In summary, the adjuvant system AS0 exerts its effects through a variety of mechanisms, depending on the ingredients used in the formulation. Use adjuvant combinations that are already in preclinical or clinical trials to create a reasonable combination to maximize effectiveness while ensuring that acceptable tolerability and safety are in place to achieve successful product development. Nevertheless, the road to obtaining permission is long, arduous and challenging, and we hope to shorten it in the future based on the lessons learned. We believe that human research using systems biology methods will continue to produce key lessons, especially those that focus on challenging the hypotheses of mechanism of action emerging in small animal research.
Cytosine phosphate guanosine 1018
TLR9 has three types of CpG oligonucleotide ligands, which can be distinguished by different nucleotide sequence motifs and their ability to stimulate IFNα in plasma cell-like DCs ( Table 1 above ). The TLR9 agonist CpG 1018 is a 22-mer unmethylated CpG-B oligonucleotide. It is an effective TH1 cell adjuvant and can stimulate strong B cell and NK cell activation. CpG 1018 is currently being evaluated as a potential vaccine adjuvant for the COVID-19 vaccine in clinical trials (see Table 2 above )). In addition, the molecule is a component of Heplisav-B, which is an improved HBV vaccine that has been approved for use in adults (age> 18 years).
Although there are other HBV vaccines that have been licensed and are widely used, they are usually given in a three-dose schedule, and the main advantage of Heplisav-B is that it provides a simplified two-dose schedule. In preclinical studies in mice, compared with the preparation without adjuvant or alum adjuvant, CpG nucleotides induced a significantly higher antibody response to various antigens (including the surface antigen of HBV and HBsAg). However, the expression pattern of TLR9 in mice is different from that of humans and rhesus monkeys. In mice, many cell types, including cells of the monocyte/macrophage lineage, express TLR9, while in humans and macaques, TLR9 expression is strictly limited to plasma cell-like DC and B cells, which highlights the understanding The importance of the molecular mechanism of agent action.
inally, the different immunostimulatory abilities of the above-mentioned different classes of CpG molecules seem to depend on whether they appear in monomeric or multimeric form. Therefore, monomeric CpG-B oligonucleotides localize to lysosomal-associated membrane protein 1 (LAMP1) positive endosomes and promote plasma cell-like DC maturation, but little or no IFNα is produced; however, when compounded into microparticles , CpG-B is located in the transferrin receptor 1 (TFR1) positive endosome and leads to the production of IFNα through a TLR9-dependent mechanism.
In addition, monomeric CpG-B can be easily taken up by B cells and stimulate their activation. In contrast, CpG-A, which spontaneously forms nanoparticle-like complexes due to its palindromic structure, is also located in TFR1+ endosomes in plasma cell-like DCs and stimulates IFNα production, but cannot be absorbed by B cells, which cannot Internalize larger DNA complexes, such as CpG-A. The innate mechanisms and cell types that stimulate adaptive immunity in response to CpG plus antigen immunity also seem to depend on the form of CpG.
Therefore, immunizing mice with soluble CpG-B plus antigen induces TH1 CD4+ T cells and antibody responses through a mechanism that relies on TLR9-mediated MyD88 activation in DC. On the contrary, the use of aggregated form of CpG-B plus antigen for immunization does not seem to rely heavily on MyD88 signal transduction in DC. It is believed that other innate cells have a role in sensing aggregated form of CpG and initiating adaptive immunity.
Emerging concepts in adjuvant biology
The role of adjuvant in CD8+ T cell response
Although it has been recognized that adjuvants can enhance the human antibody response to vaccination, so far, no adjuvant has shown the strength to induce antigen-specific CD8+ T cell responses stimulated by live virus vaccines such as YF-17D. . Some people believe that the initial dose and persistence of the antigen are the main determinants of CD8+ T cell response. However, the analysis of the immune response induced by the human live virus vaccine shows that both the antigen load and adjuvant signal play a key role in inducing the adaptive immune response.
In mice, several immunogens combined with a series of adjuvants can induce effective CD8+ T cell responses. In contrast, in humans, only live virus vaccines such as yellow fever and smallpox can induce very high antigen-specific effect CD8+ T cell responses and memory CD8+ T cell responses. Since these are replica vaccines, these studies emphasize that the initial viral load (antigen) determines the size of the human CD8+ T cell response. YF-17D mouse experiments show that the activation of DCs drives the induction of CD8+T cells through multiple TLRs and the resulting MyD88 signal.
In addition, YF-17D sends out signals through RNA sensors RIG-I and MDA5. Therefore, an important question that needs to be resolved is whether there is an adjuvant that can mimic the efficacy of live vaccines in inducing CD8+ T cell responses in humans. Accumulated experience has shown that subunit vaccines, even when delivered with effective adjuvants, will not induce CD8+ T cell responses in humans, at least as measured in blood. However, the combination of TLR ligands or TLR ligands with antigens can induce antigen-specific CD8 delivery+ T cell responses in mice. Antigen-specific CD8+ T cells can also pass through CLRs targeting C-type lectin receptors, such as DEC205 DCs, to promote cross-presentation of antigens to CD8+ T cells in mice. These strategies have been successfully applied to mice, but it is still challenging to transfer these results to NHP or humans, although the observed CD8+ T cell response can be achieved by enhancing the physical connection between TLR ligands and soluble antigens.
In addition, currently available adjuvants induce CD8+ T cell responses of much lower magnitude relative to viral vaccines such as YF-17D or smallpox, although CD4+ T cell responses are more easily induced by subunit vaccines. DNA-based vaccines and recombinant viral vectors have been extensively tested in the form of a prime-boost regimen. But there is still no CD8+ induced magnitude. T cell response observed with live viral vectors. Therefore, the discovery of adjuvants that can induce cell-mediated subunit vaccine immune responses remains a challenge for the future.
In this case, it should be pointed out that the latest results of COVID-19 vaccine trials using mRNA vaccines show that human CD8+ T cell response levels are high. Therefore, in the Phase I/II trial of BioNTech/Pfizer’s mRNA vaccine, the specific T cells of CD4+ T cells that produce IFNγ and CD8+SARS-CoV-2 receptor binding domain (RBD) spike protein are about every One million peripheral blood mononuclear cells (PBMC) 700 and 4 00, high responders have as many as 4000 per million PBMCs.
Consistent with this, by intracellular cytokine staining, the frequency of RBD-specific CD8+ T cells is very high, and the frequency of several vaccinators is as high as 4% of the total T cell pool of RBD-specific CD8+ T cells. The mechanism underlying this high-intensity CD8+ mRNA vaccine-induced T cell response is not yet clear, but it may be due to the activation of the innate immune system by nucleic acid sensing receptors or other mechanisms, and the antigen lasts for a period of time, possibly a few days or a week.
Finally, now improve tissue resident memory T cells (T interest RM cells), which are generally considered to be long-term residence within the tissue and provide a first-line defense against pathogens. TRM cells are positioned to respond quickly when reinfected at the barrier site. After the pathogen enters the mucosal site, the TRM cells that recognize the pathogen will be quickly activated, and stimulate neighboring cells in the local microenvironment to activate and recruit immune cells and establish an antiviral state. Our recent research in the NHP showed that the cells in the mucosal tissue of T RM induced by vaccination will induce antiviral gene expression programs in the resident bone marrow cells and CD4+ T cells after reactivation. It is worth noting that the congenital crosstalk of TRM cells significantly reduces the neutralizing antibody titers required to withstand the challenge of mucosal simian-human immunodeficiency virus (SHIV). Therefore, a major challenge in adjuvant research is to develop an adjuvant that can induce a large number of T RM cells.
Collectively, these observations indicate that the induction of effective CD8+ T cell responses in humans requires optimal adjuvant signaling and the persistence of antigens for at least a few days. Ideally, the kinetics of antigen existence and its distribution can be designed to be similar to the kinetics observed after administration of live virus vaccines. For example, the analysis of the immune response of the yellow fever vaccine YF-17D showed that activation of multiple innate immune receptors (TLR, RIG-I or MDA5) is necessary to induce CD8+ T cell responses. In addition, antigens that persist for about a week, as expected during acute viral infections, can also promote CD8+ T cell responses. Therefore, the failure of adjuvants to induce CD8+ T cell responses to inactivated vaccines in humans indicates the need for better control of antigen load, persistence and distribution. It is well known that replicating virus vaccines, such as YF-17D and smallpox, can induce acute viral infections in humans, which may promote the initiation of CD8+ T cells in multiple parts of the body, leading to a greater number of CD8+ T cell responses. Therefore, future research should focus on better defining the persistence and distribution parameters of antigens and adjuvants, and optimizing these parameters to determine the potential for effective CD8+ production.
At the forefront of adjuvant design and development
Recent advances in immunology have highlighted new cellular and molecular targets for adjuvant development.
- Adjuvants for inducing CD8+ T cells and tissue-resident memory T cells (T RM cells): develop new adjuvants to induce a large number of antigen-specific CD8+ T cells and T RM cells in humans, similar to those induced by live viral vectors Induce vaccines such as yellow fever.
- Adjuvants for non-Toll-like receptors (TLR) pattern recognition receptors (PRR): develop new adjuvants for PRRs other than TLRs, including nucleic acid sensing receptors, such as retinoic acid inducible gene I (RIG-I), Stimulator of interferon gene (STING) protein, C-type lectin receptor (CLR), and nucleotide-binding oligomerization domain (NOD)-like receptor (NLR).
- Metabolic adjuvants: Nutrient sensors, such as mTOR and GCN2, have a key role in regulating the function of dendritic cells (DC) and adaptive immunity. Small molecules targeting such metabolic regulators in DC may represent new adjuvants.
- Cell death adjuvant: It is now known that the uptake of dead cells by DC can lead to cross-presentation of antigens derived from cell cadavers to CD8+ T cells. In particular, recent work has emphasized that receptor-interacting serine/threonine protein kinase 3 (RIPK3)-mediated necroptosis can synergistically promote CD8+ T cell responses with NF-κB-dependent inflammation. In addition, recent work has shown that the auxiliary effect of MF59 in promoting CD8+ T cell responses is mainly mediated by RIPK3, a key mediator of necroptosis, but through a pathway unrelated to necroptosis.
- Epigenetic adjuvants: Recent work has shown that innate cells, including monocytes and macrophages, undergo epigenetic modification and collect memory-like stimulating properties in pathogen-associated molecular patterns (PAMP). Therefore, small molecules targeting such cells may represent epigenetic adjuvants that can stimulate specific aspects of the innate response (such as antiviral status, including interferon-stimulating genes (ISG)) high activation. This antiviral state of epigenetic reprogramming will provide resistance to multiple viruses for a period of time (maybe a few weeks or so).
Not just DC
DC has long been considered the primary cellular target of vaccine adjuvants. In fact, there is clear evidence that DC is essential for the adjuvant activity of TLR ligands. Therefore, in DC-deficient mice, the adjuvant activity of TLR ligands is severely affected. Even for non-TLR-based adjuvants, such as alum or MF59, the conditional absence of DCs or DCs subgroups can cause serious damage to the adaptive immune response. These studies clearly use DC as one of the key cell types for sensing adjuvant and regulating adaptive immunity. However, the immune system is a complex network of interacting cell types. Although DCs play a central role in innately sensing and coordinating immune responses, emerging evidence emphasizes the key role of other cell types in this process. For example, as mentioned earlier, the administration of alum and MF59 induces transcriptional changes in muscle cells, more so than in DC. In addition, the release of ATP from muscle cells induced by MF59 is critical to its adjuvant activity. In addition, in response to adjuvant-induced chemokine signals, monocytes and granulocytes are easier to detect at the site of muscle administration, and respond more effectively to in vitro stimulation. In addition, direct stimulation of B cells appears to be an equally important factor in producing a lasting plasma cell response. Therefore, chimeric mice that selectively lack TLR signals in B cells have severely impaired their ability to produce antibodies when immunized with TLR ligand plus antigen. Consistent with this, the intrinsic MyD88 signal of B cells proved to be essential for stimulating the response of antigen-specific B cells and antibodies to virus-like particles. Lymph node colonizing macrophages are also present in MF59 and QS-21. Finally, few studies have examined the effects of adjuvants on epithelial cells, although these cells often represent the first exposure to external stimuli. Therefore, future work should aim to more comprehensively evaluate the different cell types involved in adjuvant innate perception and their mechanisms for coordinating adaptive immune responses.
Not just TLR
Although the main focus of adjuvant discovery in the past decade has been to target the TLR pathway, it is now clear that other PRRs can be targeted to achieve adjuvant effects. The available receptors for the new generation of adjuvants include NLR, RIG-I-like receptor (RLR), CLR and STING ligand. It is worth noting that sending signals through most PRRs, including TLRs, can induce some local tissue and cell damage, and the DAMP released thereby seems to be a key component of the adjuvant activity of several adjuvants. For example, NLRs, the cytoplasmic sensor of bacterial PAMPs, recognize a variety of cell products, including ATP, uric acid, and K+ efflux, indicating that the activation is potentially mediated by cell damage ( see Figure 1 above ).
The ligands of the STING and RIG-I pathways are considered as potential adjuvants to stimulate robust CD8+ T cell responses in mice. For example, a recent study described that 2′,3′-cGAMP induces potent CD8+ T cells in mice, but of particular interest is the significant tumor regression observed with 2′,3′-cGAMP . The RIG-I and RLRS provide another possible mechanism for stimuli to be explored to possibly induce CD8+ T cell responses. Recently, it has been shown that circular RNA activates RIG-I in vivo and can be used as an adjuvant to induce cell-mediated and antibody responses. It will be interesting to further evaluate whether the circular RNA-encoded protein can induce a stronger and more effective CD8+ T cell response, because the expressed antigen is intracellular in order to be performed by the major histocompatibility complex (MHC) class I Effective delivery.
Metabolism, cell death and epigenetics
As mentioned above, it has become increasingly clear in the past decade that the innate immune system can not only sense microorganisms through PRR, but also sense various tissue damage and stress signals. For example, tissue damage can be caused by trauma, autoimmunity or infection, and lead to cell death and extracellular release of large amounts of DAMP, such as ATP or uric acid, or DNA or RNA fragments or high mobility group box 1 (HMGB1), which can activate DCs to Stimulate adaptive immunity. This raises the question of whether these innate activation pathways can be used to design new adjuvants. In fact, there is new evidence that the adjuvants that have been used in the clinic can indeed stimulate the immune response through these pathways ( Figure 2a below ). For example, as mentioned above, there is evidence that in mice, alum causes cell death and subsequently releases host cell DNA, which acts as DAMP in stimulating the immune response. In addition, as mentioned above, injection of the MF59 adjuvant vaccine into mice stimulates the release of ATP from outside the cells, and local injection of adenosine triphosphate bisphosphatase inhibits the immune response.
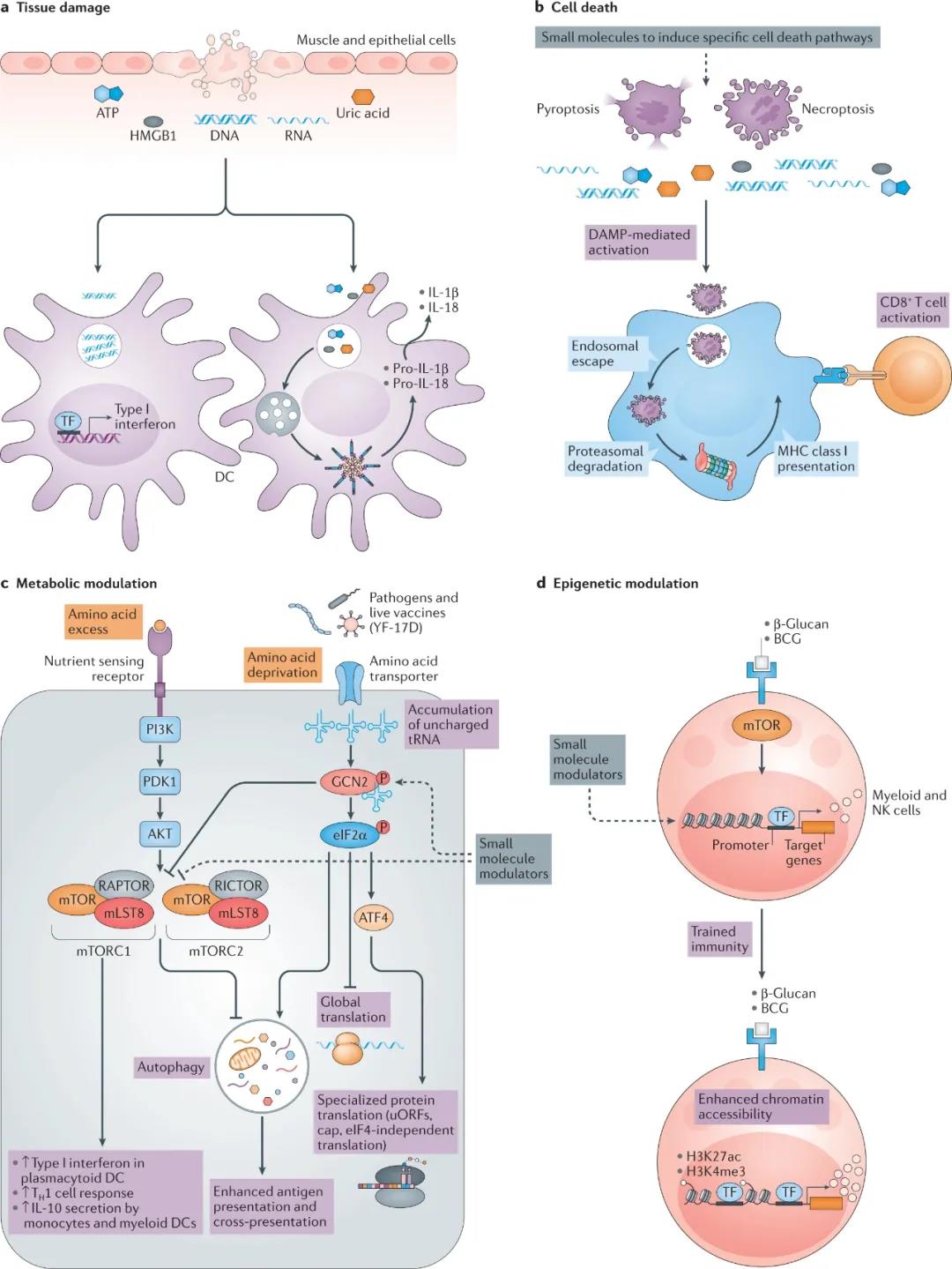
Figure 2. Other patterns of recognition receptors. Recent research has provided insight into new ways to target adjuvant activity. a. Tissue damage caused by trauma, infection and autoimmunity will cause the release of multiple damage-related molecular patterns (DAMP), including nucleic acid, uric acid, ATP and protein, such as high mobility group box 1 (HMGB1), which will activate Innate immune system. b. Cell death induced by different stimuli will also release DAMP. Of particular interest is the mechanism of cell death caused by different stimuli. Specialized cell death pathways, such as necroptosis and pyrolysis, can activate innate immune cells. Small molecules that induce specific cell death pathways may be effective adjuvants. c.
Cell metabolism is the third concept that emerged as the central regulatory network of immune response. Immune cells, such as dendritic cells (DC), have different metabolic states in different tissues. These insights stem from the systematic analysis of the human immune response induced by the yellow fever vaccine, in which the amino acid sensor GCN2 appeared as an early correlate of the long-lasting CD8+ T cell response. Yellow fever vaccine activates GCN2 in DCs and can enhance antigen presentation to T cells through autophagy.
In addition, the central metabolism regulator mTOR has been shown to have multiple effects on the innate immune response, especially DC. d. Vaccines such as Bacille Calmette-Guerin (BCG) and pathogen-associated molecular patterns (PAMP) such as β-glucan can induce epigenetic changes and keep the innate immune system on alert for a long time. Small molecules targeted to appropriate cell types provide attractive new adjuvant components. H3K4me3, histone H3 is trimethylated at Lys4; H3K27ac, histone H3 is acetylated at Lys27; MHC, major histocompatibility complex; NK cells, natural killer cells; P, phosphorylation; TF, transcription factor; TH1 cell, T helper 1 cell; uORF, upstream open reading frame.
Cell death adjuvant
Cell death has also become a key regulator of immune response. Early work has shown that foreign antigens that are not normally expected to enter the DC cytoplasm can be transferred to the cytoplasm, where they can be processed or presented to MHC class I to stimulate CD8+ T cell immunity. The follow-up work of many laboratories helped to establish this way of antigen presentation by DCs, and proved that DCs can also obtain antigens from apoptotic cells and cross-present these antigens to CD8+ T cells. However, research in the past decade has revealed many ways of cell death, and many efforts have been focused on understanding the mechanisms of necroptosis, such as necroptosis and pyroptosis. Necrosis is mediated by RIPK1, its autophosphorylation, and then recruits RIPK3, which mediates the kinase activity of necrosis. The synergistic effect of RIPK1-dependent cell death pathway and NF-κB-dependent inflammation promotes the cross-priming enhancement of CD8+T cells.
Interestingly, immunization of mice with MF59 plus antigen can cause lymph node macrophage necrosis and antigen-specific CD8+ T cells (exist in very low numbers in draining lymph nodes, but are detected more frequently in lungs and livers) Much). Interestingly, RIPK3-mediated CD8+ T cell stimulation occurs through a mechanism independent of MLKL, which indicates that RIPK3 mediates the activation of CD8+ T cells through a mechanism independent of necroptosis ( see Figure 2b above ). Taken together, these findings suggest that small molecules that can target macrophages in lymph nodes to transiently induce RIPK3-mediated pathways (including cell death) may represent adjuvants that stimulate CD8+ T cell responses ( Figure 2b above ).
Metabolic adjuvant
Another concept that has gained momentum in recent years is that the metabolic state of bone marrow cells (such as macrophages and DC) can program their innate responses and the ability to stimulate T cells. Therefore, DCs at different stages of maturity, or in different tissues, have different metabolic states. For example, the central metabolic regulator mTOR complex plays a major role in mediating TLR-induced plasmacytoid DC secretion of type I interferon, affecting the secretion of IL-12 in myeloid DC, and metabolizing reprogramming lung DC to reduce allergies. Inflammation shifts from eosinophilic TH2 to neutrophilic TH17 cell polarity, through a mechanism that leads to enhanced IL-23 and fatty acid oxidation.
In addition, the amino acid sensor eIF2α kinase GCN2 can regulate the innate function of bone marrow cells and its ability to induce T cell responses. For example, YF-17D stimulates GCN2 activation in DCs, leading to enhanced autophagy and antigen presentation to CD8+ and CD4+ T cells ( Figure 2c above ). In contrast, GCN2 leads to the inhibition of inflammasome activation, the mechanism of which involves the sequestration of reactive oxygen species (ROS), the activator of NLRP3 in autophagosomes. In addition, GCN2 changes the function of bone marrow cells by increasing the translation of the transcription factor CREB2 (also known as ATF4) and promotes immunosuppression against anti-tumor responses, which is necessary for the maturation and polarization of macrophages and bone marrow-derived suppressor cells . These results indicate that the pharmacological regulation of metabolic regulators (such as mTOR or GCN2) in DC may represent an auxiliary strategy to mimic the immune response.
Epigenetic adjuvant
Another concept that has recently emerged is innate immune memory or “trained immunity.” It has been suggested that myeloid cells, such as monocytes and macrophages or NK cells, acquire memory-like characteristics after stimulation with PAMP. This kind of “innate memory” depends on the primary stimulus, which enables the cell to be sustained epigenetic programming following the secondary stimulus or the induction of high or low responsiveness. Vaccines (such as BCG) and PAMP (such as β-glucan) induce trimethylation of histone H3 at Lys4 (H3K4me3) and acetylation of H3 at Lys27 (H3K27ac) in monocytes and macrophages (see above) Figure 2d ). These changes persist for several weeks after the stimulus is eliminated, leading to an enhanced epigenetic state.
In the second stimulation with the same or different PAMP, this enhanced epigenetic state induces enhanced gene expression. An attractive possibility is to target small molecules as pharmacological modulators to appropriate cell types to reprogram the epigenetic landscape of innate immune memory. In particular, one can imagine that small molecules targeted to monocytes or other bone marrow cells undergo epigenetic reprogramming, stimulating the high activation of specific aspects of the innate response, such as providing antiviral resistance to viruses for a period of time. Status, maybe a few weeks or so. This epigenetic adjuvant can be used to imprint an enhanced antiviral status, thereby giving humans resistance to multiple viruses. During a pandemic, such as the COVID-19 pandemic, the use of such epigenetic adjuvants may be particularly advantageous, and may enhance antiviral resistance in susceptible people for a limited period of time, which may be several weeks.
Sustained effect of adjuvant
One of the main paradigms of immunology is that the fast-reacting innate immune system senses microbial stimuli and then programs the subsequent adaptive immune response. However, emerging data also suggests that by directly targeting cells of the adaptive immune system (such as B cells), adjuvant signals have a potentially sustained effect in the later stages of the immune response. The result of immunization in the vaccine uptake by the antigen is DC, and its activation by adjuvant is contained in the vaccine, the passage of TLR or other PRR (see Figure 3 below ). Activated DC present antigens to naive antigen-specific CD4+ T helper cells, which are present in T cell-rich areas of lymphoid organs. The size and type of T helper cell response induced to a large extent depend on the nature of the adjuvant and the PRR triggered.
The activated T helper cells migrate to the interface between the B cell follicle and the T cell area, where they stimulate the clonal expansion of antigen-activated B cells. These activated antigen-specific B cells migrate to the myeloid cord in the lymph nodes and differentiate into short-lived plasma cells. Other activated B cells migrate into B cell follicles to form GC. In addition, some helper T cells upregulate BCL-6, which induces the expression of CXCR5 and CCR7, allowing migration to the B cell boundary of T cells, where they interact with antigen-specific B cells.
These TFH cells provide IL-21 and CD40L signals, which are essential for B cell proliferation and GC cell or plasma cell differentiation. According to the amount of peptide MHC class II antigen presented by B cells to T FH, antigen-specific B cells compete with each other for the help of T FH cells, providing cytokines and costimulatory signal cells at the T cell-B cell boundary. In turn, signals from B cells, including CD40L, IL-6, IL-12, and IL-23, stimulate the development of TFH cells and their migration to the newborn GC, where they play a central role in coordinating the development of memory B cells and LLPCs.
Therefore, TFH cells represent an attractive target for adjuvant design; in particular, in order to gain a deeper understanding of DC subsets and PPR on such subsets, these subsets need to be triggered to induce enhanced TFHCell response. In this case, our recent work in NHP showed that a synthetic TLR7/TLR8 ligand formulated in nanoparticles or alum, called 3M-052, can induce very high T FH cell responses and GC responses, as well as significant Persistent bone marrow LLPCs, which lasted at very high levels for 70 weeks, this was the termination point of the study. Based on these results, 3M-052 formulated with alum is currently being evaluated as a vaccine adjuvant for human HIV Env antigen (ClinicalTrials.gov NCT04177355).
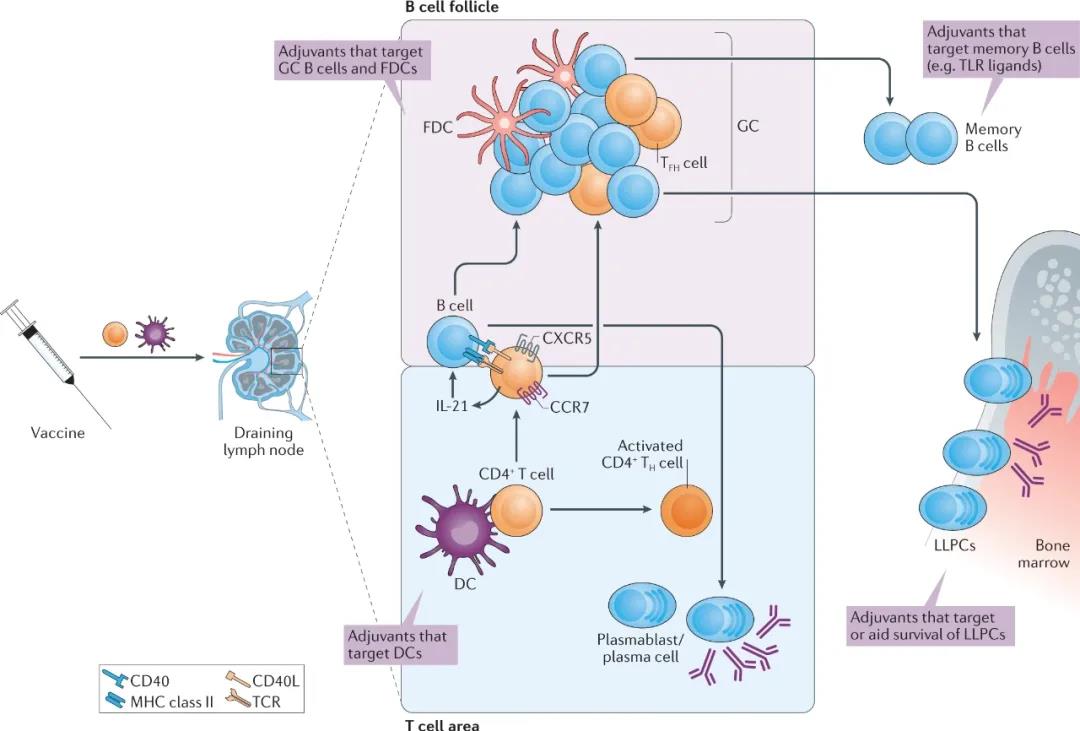
Figure 3. Beyond innate/adaptive immunity, adjuvant assistance continues to work. The innate immune system senses adjuvants and programs the subsequent adaptive immune response is the current model of how adjuvants work. Activation of dendritic cells (DC) to present antigen to T cells of naive antigen specific region CD4 + T helper cells (T H cells). Some activated TH cells upregulate CXCR5, and CXCR5 mediates their migration to the interface between B cell follicles and T cell regions, where they express IL-21 and CD40L, stimulating the clonal expansion of antigen-activated B cells.
Although some antigen-specific B cells migrate to the myeloid cord and differentiate into short-lived plasma cells, other activated B cells migrate to B cell follicles to form germinal centers (GC). The B cells in the GC can then differentiate into recycled memory B cells, or long-lived plasma cells (LLPC) that migrate to the bone marrow. Many adjuvants are known to act mainly by targeting DCs to induce their activation and antigen presentation, but emerging studies have shown that adjuvants such as Toll-like receptor (TLR) ligands can also target B cells. MHC, major histocompatibility complex; TCR, T cell receptor.
Although targeting specific DC subpopulations through specific PRR in the early “innate” stage of the immune response is a recognized adjuvant mechanism, emerging evidence suggests that even during GC, adjuvants can be B cells The response provides “continuing education” development stages. In GC, activated antigen-specific B cells proliferate rapidly, and somatic hypermutation occurs in the genes encoding their immunoglobulin receptors. As a result, new specificities of B cells have been created, including those with enhanced ability to bind immune antigens.
GC B cells compete for antigens expressed as immune complexes on follicular dendritic cells (FDC), preferentially select those cells with the highest affinity for antigens, and differentiate into memory B cells or LLPCs that migrate to the bone marrow. This process leads to “affinity maturation” of the antibody response. Activated B cells express TLR and can respond to TLR stimulation. The combination of specific TLRs can drive a persistent GC response, and as mentioned above, the inherent MyD88 signal of B cells is crucial to stimulate antigen-specific B cell and antibody responses.
In addition, TLRs expressed by fixed-dose complexes and TLR-mediated activation of fixed-dose complexes are the key to GC differentiation and affinity maturation. Therefore, this mechanism may be the key to inducing the differentiation of high-affinity antibodies and LLPC, which is essential for inducing a sustained antibody response, which is a major challenge in vaccinology. These observations indicate that adjuvants may not only target DC in the early stages of innate response, but may also play a sustained role in affecting GC response and memory B cell differentiation.
The power of adjuvant formulations
Synthetic molecules and natural products
Although the current most successful adjuvants, included in licensed products, are mainly composed of ingredients from natural sources, we believe that this does not necessarily reflect the best way to “success” in the early 21st century. It must be admitted that the ingredients used in the most successful adjuvants were generally available in the early 1990s and before. Therefore, they were the best materials to be included in product development plans at the time because they were readily available and effective, even though their mechanism of action is usually poorly understood. However, now that innate immunity has a greater understanding of the key role of adjuvants, synthetic chemistry has facilitated the development of new molecules that can be better designed as pure agonists. Although the initial discovery of TLR agonists, searches for other novel agonists that innately activate the system soon followed. The inherent properties of these newly discovered adjuvants make them more suitable for product development than the original natural molecules that they might replace. Ideally, the newly discovered adjuvant is a small molecule, which can be designed as a pure agonist, with mechanical insight, and can adjust the level of activation to control the activation signal.
Created recipes and create newRecipe method
Because such small molecule agonists benefit greatly from particle presentation, chemical manipulation can make them suitable for formulation into a preferred delivery system with flexibility in solubility and compatibility profiles. Recent studies on animal models have emphasized the value of nanoparticle-based vaccines. Here, the physical properties of nanoparticles, such as their size and surface antigen density, can affect their immunogenicity. In addition, glycosylated antigens displayed as multimers on the surface of nanoparticles can bind to innate immune defense proteins, such as mannose-binding lectin (MBL), which helps them quickly shuttle to the FDC in the GC, thereby enhancing Antibody response. Experiments in mice and macaques have shown that the formulation can also adjust the kinetics of antigen release, which affects the strength of the antibody response.
Several formulation methods have been established for adjuvants contained in licensed products. These include insoluble aluminum salts used as adsorbents, low oil content for easy injection, oil-in-water emulsions, and liposome delivery systems. Although these systems are very different in composition, they have some important similarities. They are all made into nanoparticles, but insoluble alum adjuvants are usually stable aggregates of nanoparticles, which aggregate into particles with an average size of a few microns. Importantly, alum, emulsions, and liposomes can also serve as effective “delivery systems” for antigens and immune enhancers, including TLR agonists (most notably TLR4, TLR7, and TLR9). 3M-052 is also formulated using several different methods.
Finally, a major challenge in developing these delivery systems is translation to humans. The controlled release of antigens through polymer particles has always been conceptually attractive and has received a lot of support because there are already complete biodegradable polymers that can be used in humans. However, many challenges arose in the early stages of this approach, and despite a lot of effort, they still could not be overcome; these challenges have been summarized before. In short, most recombinant antigens are degraded during the nanoparticle encapsulation process or only partially released after encapsulation, and the capture is usually inefficient, resulting in high costs due to “waste” of the antigen. Although the performance in small animals is usually very good, due to some inherent shortcomings, there is no clear way for product development. These include the fact that particles usually require expensive aseptic manufacturing in very specialized facilities, and release and quality control assays are very challenging because of the need to recover captured antigens from the particles to be assayed and quantified.
Therefore, an example of the recent successful translation of concepts into humans is the ongoing clinical project from the initial SMIP discovery. After identifying the new TLR7 agonist, although there are preclinical data showing the possibility of using multiple delivery systems, the decision was made to clinically evaluate the molecules adsorbed on alum. The reason is that alum is mature, safe and effective, and has been well known by regulatory agencies. Therefore, the new molecule is initially presented in a “familiar” form, so all questions can be focused only on the clinical characteristics of the new compound, because it can be easily evaluated together with established safe and effective vaccines. This manufacturing method is also suitable for alum, and is used in many products, and can realize the potential of the best product presentation form, such as liquid single bottles or prefilled syringes. In addition, due to the success of AS04, this method has been established as a practical method to provide TLR agonists. Therefore, for vaccines containing new adjuvants, the previously successful product development approach has been followed. Importantly, the inherent flexibility of the SMIP method allows for chemical modification of the molecule to ensure adsorption to the alum adjuvant, enabling rapid clinical evaluation without the need to establish a new manufacturing process. Encouragingly, so far, TLR7 agonists adsorbed on alum appear to be potentially safe and well tolerated by humans.
Only include the necessary things
Ensure that each component is necessary and add a clear value, while not introducing a large number of unreasonable responsibilities, can reasonably construct an improved adjuvant. AS01 is currently the most successful adjuvant contained in licensed products. Based on the level of efficacy achieved by the product, its efficacy on varicella-zoster is >97%. Prior to the development of AS01, the key ingredients MPL and QS-21 have been identified as adjuvants, and independent exploration has been carried out for a long time. However, one insight that is crucial to the development of the AS01 adjuvant is that these two molecules together lead to a synergistic effect of innate activation. Together, these two molecules induce an innate activation pattern, and no matter what the dose level, one alone cannot induce this activation pattern. Importantly, in order to achieve this synergy, a liposome delivery system was developed that allows the co-delivery of two immune enhancers to the same immune cell population. This co-delivery cannot be achieved using established alum or emulsion methods, so the development of liposomes is completely reasonable. Importantly, liposomal formulations are also effective in reducing the potential of QS-21 to induce significant local and systemic reactogenicity. Therefore, AS01 is a good example of how strong the reason is to add additional components, but the results can be used to justify the new method.
New framework for adjuvant development
Systemic vaccinology
The established method of developing vaccines containing new adjuvants has been described as one of the slowest processes in medicine. For decades, adjuvant development has relied on systematic testing of candidate molecules in mice, advancing promising candidate molecules into NHP models, and ultimately testing in humans ( Figure 4a below ). However, among all adjuvants that show strong immunogenicity and efficacy in animal models, only a few adjuvants have been proven to be safe and effective for humans. One of the main reasons for the lack of translation from mice to humans includes their evolutionary differences about 62 million years ago, and the resulting important immunological differences (such as differences in the expression of TLR7 in DC subgroups), despite their immune systems ( Both are mice and humans have T and B lymphocytes). These main differences emphasize the necessity of using human models in adjuvant testing. Recent advances in systemic vaccinology have changed our ability to detect the immune response to human vaccination with unprecedented precision.
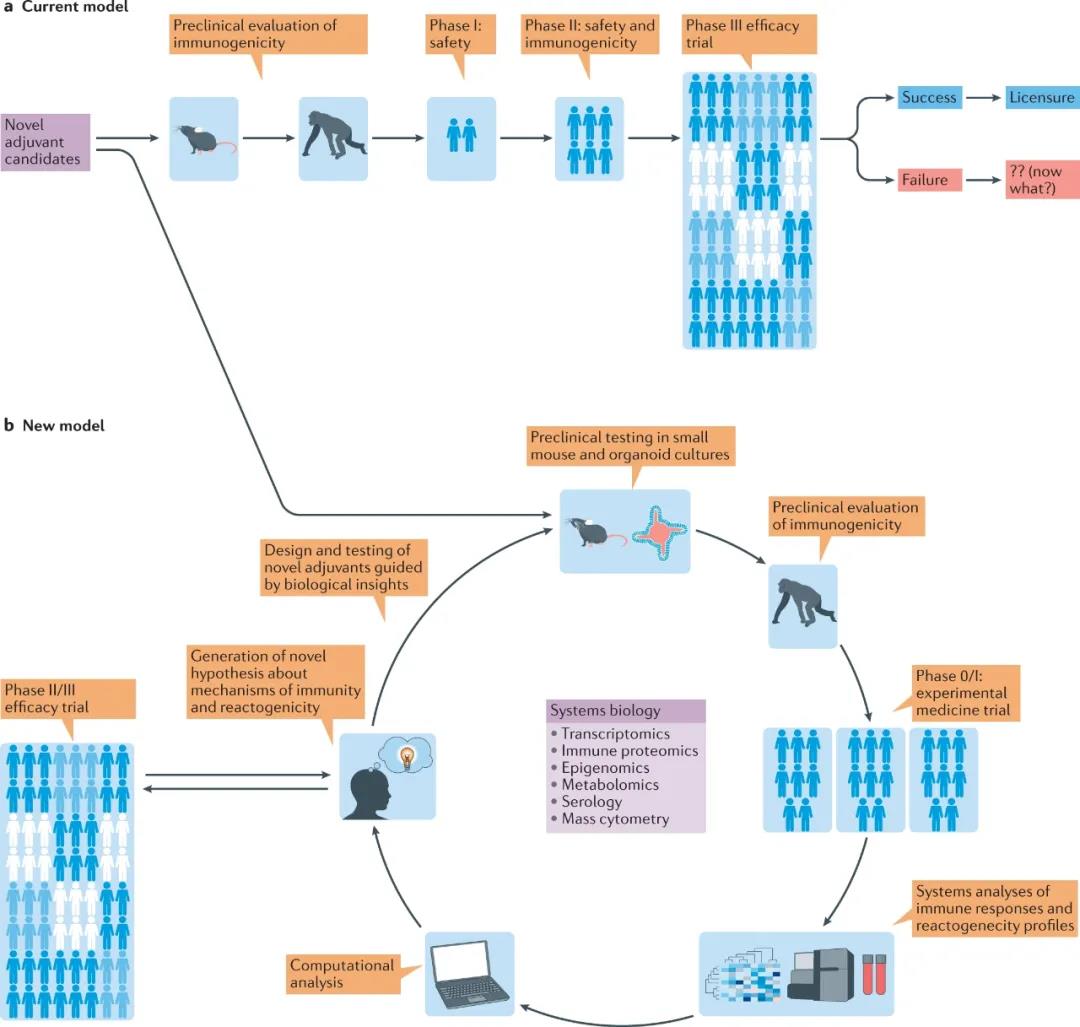
Figure 4. New framework for adjuvant development. a. The current development of vaccine models containing new adjuvants represents an advancement from the systematic testing of new vaccine candidates in mice to promising vaccine candidates tested in non-human primates (NHP), and finally The linear progression of human testing in multiple stages. b. Our new model relies on an iterative testing process on mice, organoid cultures, NHP and humans. We recommend the early use of small-scale experimental human trials and the use of systems biology methods to generate multi-parameter immunological readings, resulting in new hypotheses and adjuvant concepts that can be retested in preclinical models.
For decades, vaccine manufacturers have relied on a single method to assess the immune response to vaccination, usually serum antibody titers. One of the main limitations of this method is that it does not capture the complexity of the immune response to vaccination, so it may not be able to identify the relevance and key mechanisms of protective immunity. Systemic vaccinology uses high-throughput technologies such as transcriptomics, metabolomics, high-dimensional cell counting, and epigenomics to comprehensively analyze the immune response of vaccination, and uses the data generated by such analysis to describe the correlation of vaccine immunity Sex and mechanism.
The first study using a system-based approach to study the vaccine response determined the transcription characteristics induced within 1-7 days after vaccination, which correlated with the magnitude of the subsequent antigen-specific CD8+ T cell response and neutralizing antibody response , 25 days after the measurement. This study showed that the yellow fever vaccine triggered multiple TLRs, as well as RIG-I and MDA5, and established the innate characteristics that predict vaccine immunogenicity. Further analysis using machine learning methods proved that these features can predict subsequent antigen-specific CD8+ T cell responses and neutralizing antibody responses in an independent study of subjects receiving yellow fever vaccines. This study provides a proof of concept that systems biology methods can be used to predict vaccine immunity.
In an independent study, similar characteristics induced by yellow fever vaccines were identified, which are associated with subsequent adaptive immune responses. Based on these studies, some research groups have used this method to study the immune response to several other diseases, including malaria, meningitis and pneumococcal infections, and varicella-zoster virus. Importantly, systematic vaccinology methods have been used to identify common vaccine induction characteristics across multiple flu seasons and different populations (older people, diabetic patients). Such studies have identified characteristics related to the durability of the antibody response.
Systematic vaccinology research has produced many new insights into the mechanism of vaccine response. For example, the expression of TLR5 has been shown to be induced within a few days after vaccination and is closely related to the antibody response several weeks later. Subsequent mouse experiments showed that mice lacking TLR5 had impaired antibody responses to seasonal influenza vaccination. This may be due to the flagellin from the gut microbiota sending signals through TLR5 and providing auxiliary signals to enhance the antibody response.
Therefore, vaccination of mice treated with broad-spectrum antibiotics or sterile mice resulted in impaired antibody response to influenza vaccination. On the basis of these studies, we conducted a study in humans to evaluate the impact of the microbiota on the immune response of seasonal influenza vaccines by administering broad-spectrum antibiotics to healthy people before and after seasonal influenza vaccination. The results showed that in subjects with low pre-existing antibody titers, the loss of the microbiome resulted in the specific neutralization of H1N1 and a significant impairment of the antibody response that binds IgG1 and IgA.
In addition, the inflammatory response is enhanced (including the characteristics of inflammasome activation) and the secondary bile acid is reduced by 1000 times, which is highly correlated with the inflammatory characteristics. Multi-omics comprehensive analysis revealed a significant correlation between bacterial species and metabolic phenotypes, highlighting the key role of the microbiome in human immunity. These studies reveal the power of systematic vaccinology: first, determine the molecular predictors of human vaccine response; then, test them in a mouse model; and finally, test these mechanical insights in a new human study. This highlights the seamless continuity between human immunology research and mouse mechanism research. Other mechanistic insights from systematic vaccinology research include demonstrating the role of the amino acid sensor molecule GCN2 in regulating DC function to stimulate T cell response to yellow fever vaccine.
Advance adjuvant development
The new framework for adjuvant development may place more emphasis on testing many potential adjuvant concepts in small clinical trials (phase 0/I), as follows:
How systemic vaccinology promotes the development of new adjuvants
- Discover the mechanism of action of established adjuvants to discover new adjuvants. There is currently a lack of detailed understanding of the molecular and cellular mechanisms of the adjuvants used in licensed vaccines, including squalene-based adjuvants, such as MF59 and AS03, and saponin-based adjuvants, such as AS01b. Systematic vaccinology methods to detect the molecular network induced by such adjuvants in humans can clarify the underlying mechanism of action, which can then be experimentally verified in animal models. This detailed understanding of the mechanism can help design novel adjuvants.
- Human early stage 0/I testing to accelerate the adjuvant development process. For decades, adjuvant testing has been making progress on a linear, one-way path, first in preclinical testing in mice, and then in non-human primates. Test the most promising drug candidates in animals, and then slowly progress to human clinical testing. However, due to differences in immunogenicity between mice and humans, promising candidate adjuvants have been converted to humans. In addition, the understanding of translation failure usually occurs later in the developmental process, usually several years after preclinical research. Therefore, testing candidate adjuvants in humans in small phase 0/I trials early in the developmental process and using a system-based approach to define immunogenic characteristics will help accelerate adjuvant development. This will also help benchmark adjuvants and in combination with other successful vaccine formulations.
- Determine the mechanism and relevance of reactogenicity. A major consideration in adjuvant development is safety. Sometimes, adjuvants can cause side effects, which usually occur within a few days after vaccination, and can be local (such as erythema, swelling, or pain) or systemic (such as fever, myalgia, headache, and flu) -Like symptoms). In addition to this reactogenicity, in rare cases, adverse events may also occur, such as allergic reactions after vaccination with adjuvants. Retrospective, nested, case-control studies can be used to analyze samples from such adverse events compared with controls to determine the underlying mechanism of the host’s response to adverse events.
Therefore, new adjuvants can be quickly tested in small phase I (phase 0) human trials and systematic vaccinology methods for mechanistic insights. For example, Sékaly and colleagues studied the innate immune response of double-stranded RNA synthesized in humans (polyinosine:cytidylic acid (polyIC), and polystabilized ascending lysine (polyICLC), for the agonists TLR3 and MDA5. Transcriptional analysis of blood samples after subcutaneous injection of poly(ICLC) showed that genes involved in multiple innate immune pathways, interferon and inflammasome signaling were up-regulated in all subjects, similar to those observed with yellow fever vaccines Characteristics.
Comparative transcription analysis showed that in volunteers vaccinated with high-efficiency yellow fever vaccine, several innate immune pathways were similarly induced. Therefore, chemically defined PRR agonists such as poly(ICLC) can be a reliable and authentic Microbial mimics used to induce human innate immune responses. Similar studies can be carried out in humans vaccinated with new adjuvants that can be “benchmarked” against successful vaccines. In addition, systematic vaccinology research can be in-depth Understand the mechanism of action of adjuvants in the human body.
Even for adjuvants currently used for licensed vaccines, including squalene-based adjuvants (such as AS03 and MF59) and saponin-based adjuvants (such as AS01b, they do not seem to activate Typical TLR-dependent or other PRR-dependent innate activation pathways. Regarding saponin-based adjuvants, the results of a recent COVID-19 vaccine clinical trial and another saponin-based adjuvant called Matrix-M1 proved high Intensity of neutralizing antibody titers, but lack of detailed knowledge about the molecular and cellular mechanisms of such saponin-based adjuvants mediated its action231.
In addition, systematic vaccinology methods can be used not only to define the mechanism of action of adjuvants, but also to To define the underlying mechanism of formulation work, the underlying mechanism of adverse reactions occurring shortly after vaccination, and the rational design of the optimal formulation for vaccine delivery. The results obtained from such phase 0/I studies will help formulate the mechanism of adjuvants The hypothesis can then be tested in animal models or in vitro human organoid cultures ( see Figure 4b above ).
Here, we propose an improved rational design and iterative test model for new adjuvants-an interdisciplinary approach based on systemic vaccinology to accelerate the discovery and development of clinical adjuvants ( see Figure 4 above ). Based on our experience in managing new adjuvants to the commercial approval of vaccine products, we want to emphasize the value of early use of human studies.
The “omics” data generated from these studies can facilitate the formation of new hypotheses about the mechanism by which candidate adjuvants stimulate strong and durable antigen-specific T and B cell responses. These hypotheses can then be retested in animal models, and the resulting mechanistic insights can then be used to design new adjuvant concepts. This method of combining human models with the field of systemic vaccinology in the early stages of the adjuvant development pipeline has the transformative potential needed to revitalize adjuvant science.
Disclaimer of medicaltrend.org
Important Note: The information provided is for informational purposes only and should not be considered as medical advice.