mRNA vaccine: Mechanism Drug platform and Clinical prospects
- Normal Liver Cells Found to Promote Cancer Metastasis to the Liver
- Nearly 80% Complete Remission: Breakthrough in ADC Anti-Tumor Treatment
- Vaccination Against Common Diseases May Prevent Dementia!
- New Alzheimer’s Disease (AD) Diagnosis and Staging Criteria
- Breakthrough in Alzheimer’s Disease: New Nasal Spray Halts Cognitive Decline by Targeting Toxic Protein
- Can the Tap Water at the Paris Olympics be Drunk Directly?
mRNA vaccine: Mechanism Drug platform and Clinical prospects
- Should China be held legally responsible for the US’s $18 trillion COVID losses?
- CT Radiation Exposure Linked to Blood Cancer in Children and Adolescents
- Can people with high blood pressure eat peanuts?
- What is the difference between dopamine and dobutamine?
- What is the difference between Atorvastatin and Rosuvastatin?
- How long can the patient live after heart stent surgery?
mRNA vaccine: Mechanism Drug platform and Clinical prospects. MRNA-based drugs, especially mRNA vaccines, have been widely proven to be a promising immunotherapy.
The mRNA vaccine has significant advantages such as high efficiency, low side effects and low cost of acquisition, making it widely used in preclinical and clinical trials of various infectious diseases and cancers.
Technological progress has further alleviated the problems that originally hindered the development of mRNA vaccines, such as the low efficiency problems in gene translation and in vivo delivery. Technology upgrades can also greatly improve the immunogenicity of mRNA.
In this review, we have summarized detailed information about the optimization of mRNA vaccines and the potential biological mechanisms of this vaccine form.
The application of mRNA vaccines in certain infectious diseases and cancers, as well as the application prospects in diseases caused by bacterial pathogens (such as tuberculosis) are introduced.
At the same time, some suggestions are put forward for the future development of mRNA vaccines, involving storage, safety and personalized vaccine synthesis.
1. Introduction
mRNA is an intermediate genetic material, first discovered by Brenner et al in 1961. However, it was not until 1989 that Malone and others put forward the idea of mRNA-based drugs and proved that it is in cationic liposomes (N- [1-(2,3-dioxiranyloxy)propyl] -N, N, Under the package of N-trimethylammonium chloride (DOTMA), mRNA can be successfully transfected and expressed in a variety of eukaryotic cells.
In 1990, through direct injection, in vitro transcribed mRNA was fully expressed in mouse skeletal muscle cells. This was the first time that mRNA was successfully expressed in vivo, thus proving the feasibility of mRNA vaccine development. Since then, the study of mRNA structure and other related technologies have developed rapidly.
In this case, some developmental limitations caused by the instability of mRNA, high natural immunogenicity and low delivery efficiency in vivo are gradually alleviated, and now mRNA vaccines have been extensively studied in various diseases (Figure 1) .
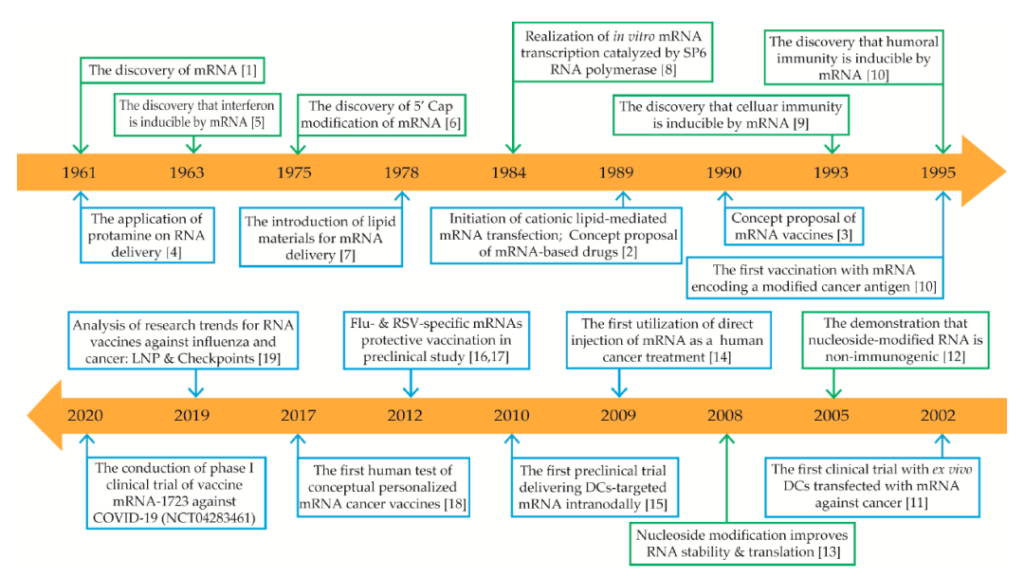
mRNA vaccines have shown many unique advantages that conventional vaccines do not have. First, mRNA can theoretically meet all genetic information requirements to encode and express various proteins. The efficiency of vaccine development can be optimized by modifying the mRNA sequence. Compared with other types of vaccine modification methods, this is a more convenient method.
In addition, although the encoded antigens are different, the production and purification processes of most mRNA vaccines are very similar. Therefore, the development of other similar mRNA vaccines may be standardized, and the use of in vitro transcription also makes the production of mRNA vaccines easier. Obviously, mRNA vaccines can save time and cost.
Secondly, mRNA has self-adjuvant properties, which can activate strong and durable adaptive immune responses through tumor necrosis factor-α (TNF-α), interferon-α (IFN-α) and other cytokines secreted by immune cells.
Peptide and protein vaccines require additional adjuvants to achieve similar goals. The in vivo expression of mRNA can also avoid contamination of protein and virus sources. By modifying the mRNA sequence and delivery system, the expression activity and in vivo half-life of mRNA can be effectively adjusted.
Third, compared with DNA-based vaccines, mRNA vaccines can express target proteins more efficiently without entering the nucleus because they are expressed in the cytoplasm.
In addition, because the chemical composition of the mRNA sequence is different from that of DNA, and the lack of CpG islands, it is less likely that the mRNA will integrate into the host DNA genome and induce immune rejection. In addition, mRNA only has transient activity, so it is easily completely decomposed through physiological metabolic pathways and will not burden the host’s homeostasis.
The first company based on mRNA drug research and development was established in 1997. Since then, a large number of companies have begun to develop drugs based on mRNA. At present, more than 20 mrna-based drug candidates have entered clinical trials.
The market for mRNA vaccines has also grown, reaching tens of billions of dollars, which means that the development of mRNA-based drugs, especially mRNA vaccines, has broad prospects.
In particular, mRNA vaccines have great potential in quickly responding to emerging epidemics, such as the global outbreak of COVID-19, which will stimulate more interest and research expectations from scientists around the world.
2. In vitro synthesis and modification of mRNA
So far, mRNA in vitro transcription technology has matured, and the most popular method is to use T3, T7 or SP6 RNA polymerase and linear DNA (linearized plasmid DNA or synthetic DNA prepared by PCR) for mRNA synthesis.
Maturation of mRNA in eukaryotic cells requires some basic structural elements, including 5’capping (5’cap), 5’untranslated region (5’UTR), open reading frame (ORF) region, and 3’untranslated region (3′ UTR) and poly(A) tail structure. Maintaining a complete structure of mRNA is beneficial to its stability and expression ability.
The mRNA sequence modified based on the complete structure can further optimize the efficiency of the mRNA vaccine. However, the initial product of mRNA in vitro transcription is a mixture of target mRNA, non-target RNA, nucleotides, oligodeoxynucleotides and proteins. In order to purify mRNA, precipitation and extraction are used to remove common impurities, and chromatographic techniques are used to separate target mRNA from other mRNA impurities.
2.1 5’ capping and modification
The mRNA of eukaryotic and part of the viral genome has a 7-methylguanosine (m7G) cap at the 5’end of the mRNA sequence, which is connected by 5′, 5′-triphosphate bridges (ppp) during mRNA in vitro transcription To the first RNA nucleotide.
5’cap can eliminate free phosphate groups in the mRNA sequence, thereby significantly enhancing the stability of the mRNA, allowing the ribosome to recognize the initiation of the mRNA by binding to the eukaryotic translation initiation factor 4E (eIF4E) and improve the translation efficiency.
Therefore, it is clear that 5’cap modification is essential to improve mRNA performance. There are two common methods for in vitro mRNA capping. The first is to add a regular m7GpppG cap analog structure to the mRNA transcription system to achieve mRNA capping and in vitro transcription. The second is in the initial stage of in vitro transcription, the capping of mRNA is completed by capping enzyme reaction.
Capping with cap analogs is the most common method for in vitro transcription of mRNA, but studies have found that conventional cap analogs can reversibly bind to mRNA sequences. In this case, mRNA isomers will be formed, resulting in low mRNA downstream translation efficiency. In order to avoid the reverse introduction of 5’cap, anti-reverse cap analogs (ARCA) have been developed. ARCA is modified at the C2 or C3 position to ensure that the methyl group reacts with the hydroxyl group at the correct position during transcription.
Compared with conventional cap analogs, ARCA capped mRNA has higher translation efficiency. In recent years, further modifications to the ARCA structure have been developed to improve mRNA properties. For example, phosphorothioate based on ARCA modification improves the translation efficiency of mRNA by increasing its affinity for eukaryotic translation initiation factor 4E (eIF4E). And by reducing the sensitivity of decapping enzymes to improve mRNA stability.
Kuhn and other studies have shown that m27,2’-OGppSpG (β-S-ARCA) can significantly improve the stability and translation efficiency of mRNA in immature dendritic cells (DC). In 2016, Strenkowska et al. synthesized a synthetic cap analog composed of 1,2-dithiodiphosphoric acid, ARCA and an extended polyphosphate chain, called “2S analog”, which has better functions than those used in clinical trials Of any S-ARCA.
Another cap analog is the co-transcription capping method developed in 2018 called “CleanCap”, which generates a naturally occurring 5’cap structure from the initial capping trimer, thereby increasing the capping efficiency to nearly 90- 99%.
2.2 Optimization of Untranslated Regions (UTRs)
UTR is the non-coding part of the mRNA sequence located in the upstream (5’UTR) and downstream (3’UTR) domains of the mRNA coding region. According to reports, UTR is related to the process of mRNA replication and translation, and can greatly change the degradation and translation efficiency of mRNA by reacting with RNA-binding protein. In order to enhance the stability and translation efficiency of mRNA, the optimization of UTR is necessary.
Generally speaking, UTR optimization is to increase mRNA expression levels in vivo. For example, the widely used 3’UTR sequences are derived from α-globulin and β-globulin that contain translation and stability control elements. It is generally believed that the 3’UTR is the region where unstable factors are concentrated in mRNA.
Therefore, avoiding unstable sequences when synthesizing 3’UTR can increase the stability of mRNA, such as AU-rich sequences and GU-rich sequences. On the other hand, the introduction of stabilizing elements into the 3’UTR can also significantly improve the stability of mRNA and prolong its half-life.
Niessen et al. found that the introduction of two random stabilizing elements in the 3’UTR successfully improved the translation efficiency of mRNA. The 5’UTR directly affects the translation of its downstream sequence ORF, so the optimization of the 5’UTR should not affect the normal translation process of the ORF.
Avoiding that the 5’UTR gene sequence is the same upstream of the ORF can effectively prevent the wrong start and reading frame replacement during mRNA translation. In addition, certain specific sequences can be added to the 5’UTR to enhance the stability of mRNA and the accuracy of translation.
For example, Kozak et al. inserted the sequence GCC-(A/G)-CCAUGG into this region to make the beginning of the translation process more accurate. Studies have also shown that the excessively stable secondary structure of the 5’UTR will hinder the binding of ribosomes to mRNA, while the short and loose 5’UTR is more conducive to the process of mRNA translation.
2.3 Codon optimization of open reading frame (ORF)
As the coding region of mRNA, the translatable rate of ORF region is very important. Therefore, choosing appropriate codons in this region can optimize the overall translation efficiency of mRNA. Optimized ORF sequences usually contain synonymous frequent codons and/or tRNA more abundant codons to replace rare codons in ORF, so that highly expressed genes can be translated using the same codons as the host, and/ Or ensure the abundance of tRNA when expressing foreign mRNA.
However, the high translation rate of mRNA is not all beneficial, because some proteins require low translation rates to fold correctly, stably and efficiently. In this case, the use of low-frequency codons in ORF can produce higher quality protein products. Therefore, for different antigens, different codon optimization strategies should be used to increase the translation rate of mRNA and at the same time ensure the quality of the expressed antigen.
2.4 The stability of Poly(A) tail and mRNA
Poly(A) tail and 5’Cap structure are both key elements in the process of mRNA translation. Poly(A) sequence can slow down the degradation process of RNA exonuclease, thereby increasing stability, prolonging the half-life in vivo and improving the translation efficiency of mRNA.
In addition, Poly(A) binding protein (PABP) can be connected to 5’Cap through translation initiation factors (such as eIF4G and eIF4E), thereby affecting the closed-loop structure of mRNA and coordinating the stability and translation efficiency of mRNA. However, PABP can also bind to adenylate complexes and participate in the process of translation inhibition mediated by microRNA.
The contradictory functions of PABP indicate that different Poly(A) sequence lengths can affect mRNA translation efficiency differently. There are many ways to synthesize Poly(A) structure. Among them, in vitro transcription using a DNA template with Poly(A) structure information can generate a definite Poly(A) sequence.
Poly(A) is added with recombinant Poly(A) polymerase by enzymatic polyadenylation after mRNA transcription to obtain a mixture of poly(A) structures of different lengths. Early studies have shown that longer Poly(A) sequences can improve the stability of mRNA.
For example, the optimal length of the Poly(A) sequence in DC is about 120-150 nucleotides, while the length of the Poly(A) sequence in human primary T cells is more than 300 nucleotides. mRNA stability and translation efficiency. When the length of Poly(A) sequence is less than 20 nucleotides, it will reduce the translation efficiency of mRNA.
However, in 2017, through a new genome-wide research technology, Lima et al. found that mRNAs with high translation efficiency usually have short Poly(A) sequences, and short Poly(A) sequences are usually found in highly translated eukaryotic mRNAs. structure.
Therefore, the above indicates that since the length of the Poly(A) sequence required for high translation efficiency mRNA is different in various types of cells, adjustments should be made to optimize the translation efficiency of mRNA.
3. Regulation of mRNA immunogenicity
Based on its own adjuvant efficacy, when mRNA is used as a carrier for foreign genes, it can show certain characteristics similar to mRNA viruses. In this case, mRNA can be recognized by antigen-presenting cells (APC) and subsequently activate pattern recognition receptors (PRR), such as Toll-like receptor 3 (TLR3), TLR7 and TLR8 [30,53,54].
Double-stranded RNA (dsRNA) can bind to retinoic acid-inducible gene I (RIG-1)-like receptor (RLR) in the cytoplasm, such as RIG-1 and differentiation-associated melanoma 5 (MDA5), and promote the maturation of APC and promote inflammation The secretion of sex cytokines and the secretion of type I interferon (IFN).
Ultimately, this leads to a strong antigen-specific humoral and cellular immune response (Figure 2). However, subunit vaccines composed of peptides or proteins usually cannot activate PRR. Therefore, it is necessary to add adjuvants. These adjuvants can initiate and support the adaptive immune response, so that the body’s immune response to subunit vaccines can achieve the desired results.
Therefore, the strong adaptive immune response and self-adjuvant properties of mRNA make mRNA show great advantages in vaccine use. Single-stranded RNA (ssRNA) can be recognized by TLR7 and TLR8 to trigger the antiviral activation state of DC during the process of mRNA transmission in the body. dsRNA contaminants can also trigger immune activation through TLR3 recognition.
However, the immune response stimulated by mRNA in the cytoplasm will stimulate cells to secrete a large amount of type I and other interferons. These interferons will inhibit the translation of mRNA and eventually lead to translation stagnation, RNA degradation, and reduced CD8 (cluster of differentiation 8) + T cell activation. Eventually the immune response ceased. This may have a negative impact on certain mRNA applications, such as vaccines and protein replacement therapy.
The self-adjuvant nature of mRNA has both advantages and disadvantages in the application of mRNA vaccines. Therefore, it is necessary to form mRNA immunogenicity regulation according to different medical needs, so as to effectively improve the application efficiency of mRNA vaccines.
Figure 2 mRNA in vitro transcription and innate immune activation
3.1 mRNA purification can adjust the self-adjuvant function
In vitro transcription products of mRNA often contain dsRNA contaminants. dsRNA is an intermediate of RNA virus genome replication and can promote the production of type I IFN. Therefore, purification of mRNA synthesis products in vitro can effectively reduce the type I IFN immune response of mRNA vaccines and improve the efficiency of mRNA translation.
Studies have shown that chromatography (rapid protein liquid chromatography, high performance liquid chromatography, etc.) can effectively remove dsRNA in mRNA products. After purification, the mRNA translation level of primary cells can be increased by 10-1000 times, and the level of cytokine secretion remains relatively high. High level.
3.2 Optimize mRNA sequence to regulate its own adjuvant properties
ssRNA can also be used as an effective pathogen-related molecular model (PAMP), causing a strong immune response and stimulating the production of type I IFN. Type I IFN can induce many types of IFN-stimulating genes (ISGs) to inhibit mRNA translation.
For example, IFN-induced proteins with quadruple repeats (IFIT) can bind to the 5’Cap structure or interact with eIF3 to disrupt the mRNA translation process. Therefore, optimizing the mRNA sequence can regulate the ability of the mRNA vaccine to activate the immune response.
PRR can recognize Cap0 (m7GpppN) blocked or unblocked mRNA and inhibit its translation. In 2014, Kumar et al. evaluated the ability of PRR to recognize three forms of capped mRNA, including Cap0 cap, Cap1 (m7GpppNmN) cap, and capless mRNA, and found that Cap1 capped mRNA can still be translated after being recognized by PRR, while Cap0 cap The uncapped and uncapped mRNAs cannot. Therefore, choosing a proper 5’Cap structure can avoid excessive immune response.
Modification of ORF region can also reduce the strong immune response caused by PRRs recognition and increase the level of mRNA translation. In 2011, Anderson et al. studied the difference between unmodified mRNA and pseudouridine-modified mRNA, and assessed that mRNA is 2′-5′-oligoadenylate synthase (OAS protein, induced by type I IFN) Recognition ability and mRNA stability, the results show that mRNA modified by pseudouridine has a lower efficiency in OAS activation, lower RNA degradation rate, and higher mRNA translation efficiency.
Karikó et al. found that intravenous injection of pseudouridine modified mRNA, compared with unmodified mRNA, found that the target protein expression in the spleen was higher and the serum IFN-α concentration was lower.
Uracil analogs are the most common analogs used for mRNA modification, and some other base analogs can also be used for mRNA sequence modification. Kormann et al. used different ratios of 5-methylcytidine and 2-thiouridine to modify the mRNA sequence, thereby effectively reducing the recognition rate of PRR and improving the intracellular stability of mRNA
3.3 Add adjuvants to optimize mRNA immunogenicity
Some studies need to enhance the immunogenicity of mRNA vaccines, and adjuvants must be added to the mRNA vaccine system to meet this demand. The traditional adjuvant MF59 (Novartis) and cationic nanoemulsion (CNE) are used to formulate RNA vaccines, which can be shown to enhance the immunogenicity and efficacy of the vaccine in different animal models.
Certain immunomodulatory molecules also have adjuvant activity. TriMix is a new adjuvant developed by the Free University of Brussels. It consists of CD3 encoding three immune activating proteins, CD70 ligand (CD40L) and TLR4 with continuous activity.
TriMix mRNA can increase the immunogenicity of naked, unmodified, and unpurified mRNA, and is also related to the maturation of DC and the enhancement of cytotoxic T lymphocyte response.
In 2018, Leal et al. used the TriMix unmodified mRNA strategy to treat patients with acquired immunodeficiency syndrome (AIDS). Treatment with high doses of TriMix mRNA showed that high HIV-specific T cell responses can be stimulated and detected. This study proved the high safety and tolerability of this strategy.
Some mRNA drug delivery vehicles also have adjuvant effects, such as cationic lipids and protamine. In 2013, researchers used cationic lipid 1,2-dioleoyl-3 trimethylammonium propane/1,2-dioleoyl-sn-glycero-3-phosphoethanolamine (DOTAP / DOPE) as an adjuvant The mRNA vaccine immunization strategy found to activate more inflammatory cytokines, and the secretion of type I IFN is also higher than the mRNA in the control DC.
After subcutaneous injection of this mRNA vaccine in mice, a large amount of type I IFN secretion and rapid aggregation of inflammatory monocytes can be detected in the lymph nodes temporarily.
This indicates that cationic lipids can enhance the adjuvant effect and efficacy of mRNA vaccines to a certain extent. Studies have also shown that mRNA and protamine complexes act as danger signals in T-help 1 cell (Th1) responses involving TLR7 and TLR8.
The RNActive® vaccine platform designed by CureVac uses co-delivered RNA and protamine complex as an adjuvant to induce Th1 T cell responses, and uses naked, unmodified and sequence-optimized mRNA as an antigen to develop mRNA vaccines.
Technically, RNA made from protamine is only used as an adjuvant, not as an mRNA carrier, so that more RNActive® vaccines can cause a strong immune response in many preclinical models, which can successfully prevent various influenza viruses. Strain attacked.
Kowalczyk et al. revealed that the treatment of mice with RNActive® vaccine can initiate a balanced and strong specific immune response through intradermal immunization. This immune stimulation only exists in the stimulated parts and lymphatic organs, and no pro-inflammatory is detected in the serum. factor. In general, RNActive® technology is a new type of mRNA vaccine technology with high safety and flexibility.
4. mRNA delivery system
mRNA needs to enter the host cytoplasm in order to express its main function specific antigen. However, mRNA molecules are not small enough to pass through cell membranes through free diffusion. In addition, both the mRNA and cell membrane are negatively charged, which increases the difficulty of mRNA delivery. In addition, the extracellular ribonucleic acid present in the skin and blood can easily degrade mRNA.
Therefore, delivering mRNA to a sufficient number of cells with a sufficiently high level of translation is one of the most difficult application problems for mRNA vaccines, because it requires a highly specific and efficient mRNA delivery system. A variety of mRNA delivery methods and mRNA delivery vehicles have been developed and applied (Table 1).
Table 1 Examples of mRNA delivery systems

4.1 Naked mRNA delivery system
4.1.1 Direct injection of naked mRNA
Early studies have shown that injection of naked mRNA can cause an immune response in mice. At present, mRNA administration methods generally include subcutaneous injection, intradermal injection, intranodular injection, intramuscular injection, intravenous injection, intratumor injection, etc.
These methods help to stimulate antigen presentation and initiate immune response. In 2013, Phua et al. found that the delivery efficiency of subcutaneous injection of naked mRNA in mice was even higher than that of mRNA nanoparticle delivery methods.
Van Lint et al. found that intratumoral injection of tumor-related mRNA will cause a moderate immune response, and believe that this may be a promising vaccination strategy in the future. At this stage, direct injection of naked mRNA is mainly used to treat or prevent infectious diseases.
However, even if injection of naked mRNA can cause an immune response, the effect of this delivery method is relatively weak, and naked mRNA usually degrades rapidly after injection.
Direct injection of naked mRNA is too simple and primitive to be used in human patients. This route of administration is often used to inject modified mRNA vaccines with other delivery systems to achieve better vaccine effects.
4.1.2 Physical delivery of naked mRNA
With the help of common physical methods such as electroporation, gene gun, and microneedle, the efficiency of naked mRNA antigen presentation can be improved. Electroporation can improve the delivery efficiency of mRNA without the need for other model receptors, which can reduce unnecessary immune responses.
Electroporation also has an auxiliary effect, which can recruit pro-inflammatory cells and induce the production of cytokines at the inoculation site, thereby improving the immunogenicity of mRNA.
In 1987, Callis et al. found that electroporation can be used to transfer mRNA into animal and plant cells with low transfection efficiency, but the product expression level in the target cell is sufficient for detection. In 2017, the mRNA transfection efficiency of electroporation in DC has reached 50-90%.
The gene gun method uses compressed helium as an acceleration force to push the mRNA coated on the surface of the gold particles into the host cell, which is an effective method of mRNA delivery.
In 1996, Qiu et al. used the gene gun method to transfer human α-1 antitrypsin mRNA into mouse skin and successfully triggered an antibody response. Peking University has developed an mRNA-based treatment method for hereditary skin diseases.
Through gene gun delivery, mRNA is effectively delivered to the target skin layer of mice. Despite the progress, the gene gun method is rarely used in large animals and humans.
The physical method of delivering mRNA may affect the physiological structure and activity of cells, and even lead to abnormal cell death. Therefore, the application of physical methods to deliver mRNA in the human body is potentially dangerous.
4.2 External loading of DCs delivery system
Dendritic cells are one of the most effective antigen presenting cells in the immune system. They can present processed antigens to CD4 +, CD8 + T cells through the major histocompatibility complex (MHC), thereby triggering cellular immunity . At the same time, DC can also present complete antigens to B cells, thereby triggering humoral immunity.
The common method of using DC as an mRNA delivery vector is to transfect mRNA encoding peptides, proteins or other antigens into DCs in vitro, and then transfer the processed DCs back to the host to initiate an antigen-specific immune response.
The DCs-mRNA delivery system does not need to be combined with other carrier molecules, can produce high delivery efficiency, and is widely used in preclinical experiments, animal models and clinical research.
In addition, this strategy is mainly applied to cancer treatment because it mainly causes cellular immune response. However, only through the endocytosis of DCs, the transfection rate of mRNA is very low, and electroporation can usually further increase the transfection rate of mRNA.
Gay et al. used electroporation to transfer mRNA encoding HIV antigen to DC for HIV treatment. After intradermal injection, the number of HIV-specific CD28 + / CD45RA-CD8 + factors/cytotoxic T lymphocytes (CTL) was at least higher than that of the control 2 times higher, enhances the T cell immune response of HIV patients.
Another obstacle that cannot be ignored in the clinical application of DC mRNA vaccines loaded in vitro is that the time-consuming and costly production process cannot meet the large demand for mRNA vaccines for certain treatment methods. In addition, in the time-consuming in vitro preparation process, the immune response caused within a few hours after mRNA transfection may be lost, resulting in a reduction in the therapeutic effect of the mRNA vaccine.
Based on these considerations, diseases that require a large amount of mRNA vaccine treatment in the short term should be given priority to a delivery system that can be produced quickly. It is also possible to consider delivery systems that target mRNA directly to APC in vivo.
4.3 Delivery system made of protamine
Protamine is a basic cationic protein with a resin-like structure. Combining mRNA and protamine in different mass ratios can produce electrostatic protamine-mRNA composite particles with different diameters. This combined form can effectively protect mRNA from the degradation of serum RNase, and the complex can cause immunity Strong immune response of cells (such as DC, monocytes, B cells, natural killer cells and neutrophils).
This indicates that protamine can be used not only as an mRNA carrier, but also as an immune activator. In 1961, protamine has been studied as one of the delivery materials of long RNA. Fotin-Mleczek used protamine as a delivery medium in the mRNA tumor vaccination process to successfully trigger a complete and specific anti-tumor response.
When the mass ratio of protamine to mRNA is 1:2, the size of the electrostatic complex formed is about 300 nm, which is relatively stable and can produce strong immune stimulation and high cytokine levels, but the disadvantage is obvious Inhibit protein expression; when the mass ratio of protamine to mRNA is 1:4, compared with the mass ratio of 1:2, protein expression increases, but the level of cytokines decreases.
Therefore, the initial consideration is that mRNA translation efficiency and immune strength are limited in the mRNA delivery system formulated with protamine, and it is speculated that this defect may be related to the extremely tight electrostatic complex.
In recent years, protamine mRNA delivery systems have been widely used in clinics and have achieved good clinical treatment effects, such as rabies, non-small cell lung cancer and so on. The RNActive® vaccine platform that only uses protamine-mRNA to activate the immune response is a popular technology to solve this problem.
In addition, to use protamine as an mRNA delivery and immune activator at the same time, the structure optimization of protamine or the search for proteins with similar properties to protamine as substitutes deserve our attention.
4.4 Cationic liposome delivery system
As a commonly used gene carrier, cationic liposomes can also combine with negatively charged nucleic acids to form electrostatic complexes, thereby improving the delivery efficiency of mRNA.
Cationic lipid-mRNA complexes and other preparations can form 80-200 nm nanoparticles, called lipid nanoparticles (LNP), which can be transfected into the cytoplasm through endocytosis.
LNP is one of the most advanced mRNA delivery systems. One. This stable particle is composed of ionizable cationic lipids, natural phospholipids, cholesterol and polyethylene glycol (PEG).
Ionizable cationic lipids can promote the autonomous aggregation of mRNA to form 100 nm particles, and release mRNA in the cytoplasm through ionization; natural phospholipids support nanoparticles to form a lipid bilayer structure; cholesterol is used as a stabilizer to increase the stability of LNP ; PEG can extend the half-life of the LNP complex.
The mRNA is carried in the core of LNP, which can protect it from degradation. The lipophilicity of the LNP material allows the mRNA delivery complex to fuse with the host cell membrane and deliver the mRNA to the cell through endocytosis. In early research, LNP was often used as a short interfering RNA (siRNA) delivery system. Today, LNP is also widely used in the process of mRNA delivery.
Geall used LNP to deliver the RNA vaccine to make the mRNA expression level of mice significantly higher than that of the naked mRNA group, and can effectively induce CD4 + and CD8 + T cell immune responses.
Under different routes of administration, the immunostimulatory area stimulated by LNP-mRNA may be different, and can achieve the needs of targeted therapy for different diseases.
Pardi found that subcutaneous, intramuscular, and intradermal injections of appropriate doses of LNP-mRNA can mediate the expression of local gene products.
Research in 2018 showed that intravenous injection, intraperitoneal injection, tracheal inhalation and other LNP-mRNA treatments can achieve the systemic expression of gene products. Among them, intravenous injection showed the highest mRNA delivery efficiency, and the target protein product was successfully expressed in the liver on day 4. .
However, it is worth noting that the escape mechanism of mRNA from the complex to the dissociated state to function in the cytoplasm is still not fully understood.
The change of the ionization state of lipids with the pH of the environment in the body is considered to be essential for the escape process. At the same time, further research is needed based on the reduction of toxicity and immune regulation of cationic lipid delivery systems.
4.5 Polymer-based delivery systems
At present, cationic polymers have been widely used as mRNA delivery vehicles. Commonly used polymer transport materials include polyethylene imine (PEI), poly (β-amino ester) (PBAEs) and so on. Among them, PEI is one of the most widely used materials. PEI is a cationic water-soluble polymer with a dendritic, linear or branched structure, mainly used as a DNA/mRNA carrier.
The commercial linear PEI derivative jetPEI™ has been used for DNA and siRNA transfection, and it is currently available for mRNA transfection. However, PEI has certain cytotoxicity and is difficult to degrade, so researchers often use fatty chains to modify low molecular weight PEI to reduce PEI toxicity. PBAEs are biodegradable polymers originally developed for DNA transfection.
A 2007 study showed that PBAEs can be used to deliver mRNA, and when there is no serum protein in the system, higher levels of in vitro mRNA transfection can be achieved.
This research has led to the development and application of a variety of PBAEs that enhance serum stability in vivo. Due to the simple synthesis method of PBAE, thousands of PBAEs with different chemical composition have now been formed. In addition, PBAE and lipids can be formulated together to improve their serum stability.
In 2016, Kaczmarek et al. developed a polymer-based delivery system by formulating PBAEs and lipid-PEG together. The system has high serum stability and mRNA delivery efficiency, and successfully detected small amounts of small cells by intravenous injection. Target mRNA product in mouse lung.
Polymer-based materials are strong competitors for lipids in mRNA therapeutics. Their toxicity is similar to cationic lipids, which also prevents them from being widely used. In addition to modification with other materials to improve the performance of polymer-based carriers, the optimization of molecular weight and branching seems to be a reliable direction.
5. Application of mRNA as a drug platform
Immunotherapy, especially vaccines against infectious diseases and cancer, is the core area of the mRNA drug platform application. Research in other fields, such as reprogramming of cell fate and mRNA-based genome editing, has published many reviews, so these are no longer the core of concern in this review.
According to the construction and replication ability, mRNA vaccines are generally divided into two main types: self-amplifying mRNA (SAM) vaccines and non-replicating mRNA vaccines.
The self-amplified mRNA vaccine is developed from the alpha virus genome, and its structural protein sequence is replaced by the antigen sequence that we want. Due to the integrity of the virus replication mechanism, it can achieve intracellular RNA amplification and a large number of antigen proteins. expression.
The full length of naked SAM can reach 9-10 kb. Due to self-replication, the low-dose use of this vaccine is expected to produce a large amount of antigens and has a fairly long validity period (up to 2 months).
The vaccination of self-amplified mRNA vaccines can mimic acute pathogen infection due to its PAMP, the replication of the RNA encoding the antigen from the adjuvant, and the protein expression that occurs within a few hours after vaccination.
However, this feature of self-amplified mRNA vaccine is still controversial, because it may limit the size of the antigen coding sequence that can be accommodated, affect the accurate regulation of the induced inflammatory response, and even cause the organism’s immune response to those RNA replication factors. Thereby reducing the effectiveness of the vaccine.
Non-replicating mRNA vaccines have the complete structure of mature mRNA, which contains ORF fragments encoding our desired antigens. Due to their short length (2-3 kb), there is no limit to the load on the antigen, which can better control the triggered immune response and develop a cheaper method from synthesis to storage. Non-replicating mRNA vaccines have great potential and can be the main cure for current epidemic outbreaks.
As mentioned earlier, research on mRNA vaccines has largely completed the establishment and preliminary exploration of the concept in the 1990s. In 1993, Martinon et al. successfully achieved the in vivo induction of specific anti-influenza CTLs by encapsulating mRNA encoding influenza virus nucleoprotein with liposomes or subcutaneously in mice. This is a pioneering attempt at mRNA vaccine vector.
In 1998, Mandl et al. used gene gun to deliver infectious RNA synthesized in vitro from flavivirus, and proved that less than 1ng of RNA can induce protective immunity in mice.
Boczkowski et al. found that DCs obtained enhanced antigen presentation ability by pulsed with tumor-derived RNA in vitro, and reported anti-tumor effects in vitro and in mice.
Loading of isolated DCs to achieve targeted antigen presentation has become a highly sought-after delivery strategy for cancer vaccines to stimulate the immune response of anti-tumor cells.
In 1999, Zhou et al. directly injected glycoprotein 100 mRNA encapsulated in Japanese hemagglutinating virus (HVJ) liposomes into the spleen, and proved that the mouse melanoma model inhibited tumor growth and prolonged survival time, indicating that the mRNA vaccine has Great potential for fighting cancer.
5.1 mRNA vaccines against infectious diseases
Vaccines to prevent infectious diseases have always been the most effective way to prevent and limit infectious diseases. A typical example is the complete eradication of variola virus.
Unfortunately, traditional vaccine strategies (such as non-live freeze-dried vaccines and live attenuated vaccines) have performed poorly against certain long-term or recurrent pathogen infections (such as AIDS and tuberculosis).
Due to the relatively slow development speed, it is impossible to solve the outbreak of virulent pathogens such as Zaire Ebola virus, Zika virus (ZIKV) and coronavirus.
The mRNA vaccine for infectious diseases has achieved encouraging results, and some products have entered human clinical trials (Table 2).
The overall development steps for these vaccines are:
(1) Construct an optimized or combined core coding antigen mRNA sequence based on the target pathogen;
(2) Try and select a suitable combination of mRNA construction type, adjuvant, carrier material and route of administration;
(3) Detect the in vivo expression of the encoded antigen and the level of immune response caused;
(4) Provide research and demonstration of immune induction mechanism. Here, we review some recently published studies related to mRNA vaccine application trials.
5.1.1 Influenza virus
Influenza viruses have the characteristics of continuous evolution, which makes it difficult to completely eradicate them. Monoclonal antibody therapy against conserved sites of influenza virus effector molecules is generally considered to be a highly specific and effective method against this virus.
The mRNA vaccine encoding the conserved region of the influenza virus effector protein can stimulate the production of specific antibodies, so compared with conventional vaccines, better preventive or therapeutic effects can be obtained.
In addition, the rapid production process of mRNA vaccines makes it easier to stand out in the prevention of new influenza viruses. Current anti-influenza mRNA vaccines mostly use cationic lipid-based delivery systems to deliver mRNA. The RNActive® vaccine platform with self-adjuvant properties also has good performance in influenza prevention trials.
Brazzoli et al. developed a new type of oil-in-water CNE as a carrier for the SAM vaccine expressing influenza virus hemagglutinin (HA) antigen. According to reports, the vaccine can effectively induce the immune response of functional neutralizing antibodies, HA-specific CD4 + Th1 cells and CD8 + cytotoxic T cells. It also defends mice against the challenge of lethal influenza viruses.
Pardi et al. were immunized with a non-replicating mRNA vaccine encoding the full-length influenza virus HA delivered by LNP and successfully elicited HA stem-specific antibody responses in mice, rabbits and ferrets.
This mRNA-LNP influenza vaccine can overcome the inhibitory effect of maternal antibodies to a certain extent, thereby inducing a longer time and stronger immune protection in mouse pups than conventional influenza vaccines.
Feldman et al. reported the first phase of clinical trials of two non-replicating mRNA vaccines against influenza viruses (H10N8 and H7N9). These vaccines encode the full-length HA of H10N8 and H7N9, respectively. The mass ratio of mRNA to LNP is At 1:20, both vaccines used the LNP vector, which was first used in the mRNA vaccine against Zika virus. Healthy adults tolerated LNP well and caused a strong humoral immune response.
This study shows that mRNA vaccines can address highly variable pathogens.
5.1.2 AIDS
Since the discovery of AIDS in 1981, a truly effective and affordable treatment has not yet been found. Fighting HIV is an important subject in the research and development of mRNA vaccines. Currently, there are several mRNA vaccines used to treat AIDS in human clinical research.
Ex vivo loading of DC delivery systems seems to be the preferred delivery method commonly used for cancer treatment. In infectious diseases, it is almost exclusively used for the treatment of AIDS, and has been widely proven to safely cause antigen-specific CD4+ and CD8+ T cell immune responses.
However, the results of a clinical trial reported by Gandhi et al. in 2016 were disappointing. The trial immunized HIV-1 positive participants with autologous DCs transfected with mRNAs encoding HIV-1 structural proteins Gag and Nef. In the test, only a short and weak immune response was detected, indicating that DC vaccination needs to be improved.
In this way, the delivery system that can cause a strong antigen-specific T cell immune response is receiving more and more attention in the treatment of AIDS.
Cationic nanoparticle carrier is a diverse and promising delivery system. Zhao et al. developed a self-assembled cationic nanomicelle based on PEI-stearic acid (PSA) copolymer, which can provide a non-replicating mRNA vaccine encoding HIV-1 Gag. Their research shows that PSA/mRNA nano The micellar vaccine has the potential against HIV, has acceptable carrier toxicity, effective endosomal escape and translation of mRNA in DC, and stimulates effective secretion of specific antibodies and expression of pro-inflammatory cytokines.
Bogers et al. demonstrated that a SAM vaccine encoding HIV-1-C clade envelope glycoprotein delivered by the CNE system, including squalene, DOTAP, sorbitan trioleate and polysorbate, has relative Mature preparation program. This HIV SAM vaccine replaces the other two forms of SAM vaccine and can induce a larger cellular immune response and neutralizing antibody response. The self-amplified mRNA is wrapped by HIV recombinant envelope protein or engineered virus replicon particles.
HTI-TriMix is a combination of activating adjuvant and selected mRNA, which contains 16 conservative fragments from HIV-1 structural proteins Gag, Pol, Vif and Nef. It is a novel mRNA-based therapeutic vaccine against HIV-1 It encodes a strong activation signal and an effective HIV recombinant antigen.
Preclinical results show that the vaccine can effectively induce mature DC, antiviral cytokine secretion (especially IFN-γ) and T cell stimulation.
Mice injected with HTI-TriMix into nodules produced effective antigen-specific cytotoxic T cell responses. By the end of 2019, the phase I and phase IIa clinical trials of HTI-TriMix have been completed. In Phase IIa, participants infected with HIV-1 received 3 vaccinations at 0, 2 and 4 weeks. The vaccine was administered via ultrasound in the inguinal lymph nodes.
Although HTI-TriMix showed good safety and tolerance, unfortunately, an unexpected start codon was found upstream of the HTI recombinant antigen coding sequence, which may have a negative impact on the expression of HTI protein. The later studies of HTI correction are still uncertain. Considering other translation processes of mRNA vaccines, preliminary experiments on mRNA expression in vitro are worthy of our attention.
Due to limited understanding of HIV and unclear pathogenesis, there are still many difficulties in the treatment of AIDS. In the future, the design of mRNA vaccines should emphasize the selection of appropriate antigens and delivery systems to elicit a strong antigen-specific T cell immune response. In addition, mRNA vaccines to prevent AIDS may also be a viable area.
5.1.3 Coronavirus
In the past 20 years, three types of coronavirus infections (SARS-CoV, MERS-CoV and SARS-CoV-2) have occurred globally, all of which have caused huge health threats and economic losses. There is no mature cure for this disease. Therapies or vaccines. Up to now, among all the patents related to vaccine types, only three patents related to SARS and MERS are related to mRNA vaccines.
In the face of the new coronavirus epidemic, the speed of vaccine development determines the speed of life-saving. Therefore, mRNA vaccines with rapid production processes will play an important role in the development of coronavirus vaccines.
As of August 25, 2020, COVID-19 caused by SARS-CoV-2 infection has spread all over the world, with more than 23.51 million confirmed cases and 810,000 deaths (WHO data). There is an urgent need for an effective vaccine.
Lin et al. reported two non-replicating mRNA vaccines, respectively encoding the receptor binding domain of the spike protein and the virus-like particle (VLP) of SARS-CoV-2. The optimization of the antigen sequence and the evaluation of safety and efficacy are in progress. .
Moderna announced for the first time the mRNA candidate vaccine mRNA-1273 for SARS-CoV-2, and officially started phase I clinical trials on March 16, 2020, and conducted safety and immunogenicity evaluations. The vaccine encodes SARS-CoV-2 According to the temporary data released on May 18, 2020, mRNA-1273 has been proven to be generally safe and well tolerated.
Two weeks after the second injection, the vaccination dose was as low as 25 µg, and the levels of binding and neutralizing antibodies in the serum were the same as those detected in samples from people who recovered from COVID-19 infection. Sanofi Pasteur and Translate Bio announced the joint development of a new mRNA vaccine against SARS-CoV-2 on March 27, 2020.
Pfizer and BioNTech announced the positive results of the ongoing phase I/II clinical trials of BNT162b1, which is a modified mRNA vaccine delivered by LNP, encoding the trimeric SARS-CoV-2 S protein receptor binding domain, and initially determined the suitability of BNT162b1 The dose level is between 10µg and 30µg.
After two doses of 10 µg and 30 µg BNT162b1, the average titers of specific neutralizing antibodies were 1.8 times and 2.8 times that of the specific neutralizing antibodies in the recovery period, respectively.
5.1.4 Other viral pathogens
CV7201 is a preventive non-replicating mRNA vaccine candidate that binds to the protamine encoding the rabies virus glycoprotein (RABV-G). Phase I clinical trials were completed in 2016. In preclinical trials, this vaccine can produce a strong antibody response with a stable titer level of up to one year when injected into the skin of mice and pigs, and induce strong specific CD4+ and CD8+ T cells (compared to commercially available products). CD4+ T cells induced by chemical vaccines are more highly induced).
Although CV7201 was shown to be safe in the first phase of trials, the unstable functional antibody titers made the research prospects unclear. Subsequent new preclinical studies conducted in 2019 reported that compared with the mRNA candidate vaccine formulated with protamine, the use of RABV-G mRNA packaged in LNP improved body fluids and fluids in both mice and non-human primates. Cellular immune response; corresponding human clinical trials are also under follow-up.
In 2017, Cell and Nature respectively reported an improved mRNA-LNP vaccine against ZIKV. When ZIKV invades the endoplasmic reticulum cavity, the two ZIKV structural proteins, membrane protein (prM) and envelope (E), form a prM-E heterotrimer.
Richner et al. developed a non-replicating mRNA vaccine encapsulated by LNP encoding the human IgE signal sequence, which contains the full-length prM and E genes (IgEsig-prM-E). 2μgIgEsig-prM-E LNPs were vaccinated intramuscularly, and strong detection was detected. Effective neutralizing antibodies (> 1 / 100,000 EC50), challenge experiments show that mice can be protected from severe ZIKV infection.
Similarly, Pardi et al. demonstrated that inoculation with a low dose (50 µg) of the mRNA-LNP complex encoding the ZIKV prM-E glycoprotein can adequately protect non-human primates from virus attack.
Two synthetic and CNE-encapsulated SAM vaccines against Venezuelan equine encephalitis: LAV-CNE (RNA genome carrying TC-83, live attenuated vaccine vaccine strain) and IAV-CNE (genome carrying TC-83 virus with capsid gene deletion) ) Is designed to provide immune protection.
In the vaccinated mice, both vaccines induced strong specific neutralizing antibodies and produced protection against wild-type VEEV aerosol challenge. In addition, candidate vaccines based on mRNA have been developed and tested against various viruses such as chikungunya virus, herpes simplex virus, human interstitial pneumonia virus and parainfluenza virus, all of which have shown satisfactory development prospect.
It is worth noting that Pepini et al. found that type I interferon, which plays a key role in the antiviral response, can inhibit the expression of the mRNA-encoded antigen delivered by LNP in mice. Similarly, Zhong et al. found that the naked ZIKV SAM vaccine encoding ZIKV prM-E induced limited and unstable humoral immunity in wild-type mice compared with the strong response of IFNAR1 knockout mice.
These studies have shown that the antiviral response activated by the SAM vaccine, especially the type I IFN response, may have a negative impact on the immune protection induced by SAM, and the construction and administration of SAM should be optimized.
5.1.5 Bacterial pathogens
In addition to viral antigens, only a handful of bacterial and parasite antigens have been used in mRNA vaccine attempts, many of which are still in preclinical testing.
In 2017, Maruggi et al. designed two preventive SAM vaccines, which were combined with the CNE of group A (GAS) streptococci encoding streptococcus hemolysin-O (SLOdm) and the pilus 2a skeleton protein of group B (GBS) streptococci ( BP-2a) mixed, successfully produced a large number of specific antibodies after inoculation of mice, and the survival rate of GAS and GBS infection was improved.
Among infectious diseases caused by a single pathogen, tuberculosis caused by the bacterial pathogen Mycobacterium tuberculosis has long ranked first in the global mortality rate. There is currently only one licensed vaccine against human tuberculosis: an attenuated whole cell The vaccine, Mycobacterium bovis Bacillus Calmette-Guerin (BCG), has found serious limitations in many clinical trials. MV A85A, a TB subunit vaccine expressing a single antigen Ag85A, has no obvious protective effect in phase IIb trials.
Disappointingly, compared with viral infections, bacterial infections tend to have more complex stages, with different molecular expression characteristics, and it is actually impossible to cover a single antigen.
If you want to further apply mRNA vaccines to the field of prevention and treatment of bacterial infections, you should consider more optimizations, including the selection and recombination of various antigens or epitopes, regular administration of different target antigens, and even direct adjuvant passive immunization (For example, Kose et al. developed a chikungunya anti-mRNA vaccine encoding a neutralizing human monoclonal antibody).
We are all looking forward to the first successful mRNA vaccine product. Optimizing the primary and secondary structure of mRNA and selecting the appropriate delivery system according to the characteristics of different diseases are the key steps for better application of mRNA vaccines against various pathogens.
5.2 mRNA cancer vaccine
As our understanding of tumor-specific antigens gradually deepens, people are now discussing the possibility of developing cancer vaccines. Tumor-associated antigens (antigens that are preferentially expressed in cancer cells, usually related to the disorder and abnormal behavior of cancer cells) and tumor-specific neoepitopes (small peptides derived from tumor-specific somatic mutations, exposed on the surface of cancer cells) And can be recognized by T cells)) is now the core target of mRNA cancer vaccines.
Taking into account the diversity and uncertainty of cancer occurrence, cancer vaccines currently mainly used for treatment are aimed at stimulating cellular immunity, which may become an effective cancer treatment method. There have been a number of clinical trials on prospective candidates (Table 3).
Sahin et al. pioneered the concept of “mutant genome”, that is, the overall detection and localization of somatic mutations in tumor patients. The acquisition of next-generation sequencing technology makes individualized vaccine therapy possible and attractive.
In addition, they designed a program from mutation identification, new epitope prediction to personalized mRNA vaccine development. This makes the mRNA vaccine unique for each patient.
This strategy was first applied to patients with melanoma and achieved encouraging results. Using exome and RNA sequencing to compare tumor biopsies and normal blood cells, the researchers identified and selected ten mutations related to human lymphocyte antigen (HLA) function in each patient. Based on these mutations, core mRNA was synthesized and reported to Neo-epitope vaccine (≥8 doses) was injected percutaneously into the inguinal lymph nodes.
A strong T cell response to multiple new epitopes encoded by the vaccine was detected in all patients. Through PD-1 blockade combination therapy, some patients have a complete response to vaccination. Clinical trials of similar mutation-based mRNA vaccines against triple-negative breast cancer are ongoing.
Electroporation is usually used to co-transfect DCs with mRNA encoding immunoregulatory factors to enhance the immune response caused by DCs-mRNA cancer vaccines. In many animal experiments, TriMix can promote DC activation, CD4+ T cell phenotype transfer and cytotoxic T lymphocyte response.
Combination therapy has been combined for advanced melanoma. The therapy includes injections of DC-based mRNA vaccines encoding TriMix and tumor antigens and ipilimumab, which successfully induced potent tumor-associated antigen-specific CD8+ T cells The response proved the excellent therapeutic effect of tumor-specific vaccines and immune blockers.
However, in 3/15 patients, there are still undetectable responses after T cell stimulation in vitro, which indicates that it is necessary to further study the mechanism of related issues. In addition, Reinhard et al. developed a chimeric antigen receptor (CAR)-T cell that targets and regulates the tight junction protein claudin 6 (CLDN6), and used liposomes to deliver RNA vaccines encoding CLDN6, which greatly promoted CAR-T. Cell stimulation and regression of solid tumors in mice.
In view of the obvious uneven distribution of various lymphocytes throughout the body, and the different locations and attributes of different primary tumors, it is necessary to choose a suitable carrier and administration method to optimize the effect of the mRNA vaccine.
The above are general transportation systems and transportation routes. For example, Jabulowsky et al. developed an RNA-lipoplex vaccine against melanoma, which can stably deliver mRNA to APC throughout the body by intravenous injection to express and present antigens. This vaccine is undergoing clinical evaluation of safety and tolerability (NCT02410733).
Direct intratumoral vaccination is an emerging method. Shariati et al. pioneered pressurized intraperitoneal aerosol chemotherapy (PIPAC) for delivery of mRNA complexes and demonstrated that PIPAC can apply mRNA to the abdominal cavity of mice.
In addition, in order to optimize and select drug delivery systems, Anderson et al. developed a high-throughput method to screen suitable ionizable lipid-like materials as mRNA delivery vehicles. They used an isocyanide-mediated three-component reaction to construct a combinatorial library of ionizable lipidoid substances. The screening criteria can promote mRNA delivery in vivo and provide effective specific immune activation.
It is further proved that the selected best candidate compound heterocyclic lipid-mRNA vaccine can promote APC maturation through intracellular stimulus of the interferon gene pathway, thereby stimulating a strong immune response.
6. Discussion
As mentioned by Weissman et al., in vitro standardized production of mRNA has become possible, but there are still obstacles in synthesizing some uncommon sequences and reducing the production cost of related reagents.
At the same time, research on maintaining the activity and shelf life of mRNA vaccines should be strengthened. It was previously reported that the purified freeze-dried RNA can be stored in trehalose at 4°C for up to 10 months, and its stability is comparable to traditional vaccines.
Phase I trials of the mRNA-based rabies virus vaccine CV7201 showed that it can be stored as a freeze-dried formulation at 5-25°C for 3 years and at 40°C for 6 months without significant loss of activity. In 2019, Coolen et al. developed an mRNA vaccine based on polylactic acid-nanoparticles, mixed with amphiphilic cationic peptides, and showed stable expression after being stored at 4°C for up to 7 days.
Although further studies are needed to study the storage effect of mRNA complexed with carrier molecules under freezing conditions, studies have shown that mRNA vaccines are possible in the future for free cold chain transportation and storage.
Compared with traditional vaccines, mRNA vaccines are widely recognized mainly because of their non-toxic production process, short production time and the chemical properties of ribonucleic acid, which are consistent with the safety and tolerance of mRNA vaccines shown in a number of clinical trials.
However, various adverse symptoms of unknown cause are still occasionally discovered, which emphasizes the importance of safety optimization. Autoimmunity triggered by type I IFN response is considered to be the cause of adverse reactions. There are other problems, such as edema and coagulation due to excessive extracellular RNA and the production of anti-mRNA antibodies.
Side effects caused by the carrier or route of administration may also exist. Both safety assessment and research on the mechanism of anti-vaccine response need to be advanced.
Although no mRNA vaccine products have been approved for marketing so far, the development of a series of mRNA vaccine products should be included in the government plan, especially considering the trend and potential in this field. There are a large number of relevant preclinical studies and clinical trials in this field. In progress or completed.
The development of tools for material screening or characterization based on mRNA complexes is essential to improve the stability of mRNA vaccines and the efficiency of protein production. As mentioned above, the construction of combinatorial libraries of ionizable lipid-like substances is a promising strategy.
In 2018, Zhang et al. used fluorescence spectroscopy (FCS) to analyze the stability of mRNA-based complexes in buffers and biological fluids (such as human serum and ascites). The results showed that the strong binding of linear PEI to mRNA is likely to cause The translation efficiency of mRNA is reduced, and lipid-based carriers perform well in effective intracellular release and subsequent mRNA translation.
In addition, they used FCS and single particle tracking to study the decay kinetics of mRNA measured in biological samples and its half-life (about 1-2 minutes). The single-molecule method has attractive application prospects in constructing mRNA vaccines.
In theory, mRNA can synthesize and express almost all protein antigens, which can provide great flexibility for antigen design. For example, Richner et al. developed an mRNA that contains a mutant antigen encoding ZIKV, in which the epitope that is cross-reactive to dengue virus is deleted, in order to minimize the induced antibody cross-reaction.
Nowadays, the application of artificial intelligence and deep learning has made great progress in protein structure prediction based on gene sequence. At the same time, the development of big data has greatly promoted the advancement of tumor epitope prediction algorithms.
With the further development of the field of data mining, better optimization of existing antigens and design of unprecedented antigens independent of natural genes may become a reality. The optimization and personalization of mRNA vaccines will become a revolutionary milestone.
In short, mRNA vaccine is a multifunctional and powerful platform. Its successful clinical application has significantly enhanced our ability to respond to and control emerging infectious diseases, and from the perspective of stimulating autoimmune responses, it has significantly enhanced our ability to treat classic and re-emerging infectious diseases and cancers.
The mechanism of extracellular transport, intracellular escape, and mRNA gene expression needs to be further studied. In addition, the modular design and production of mRNA vaccines under different application conditions have broad prospects in clinical applications. In the next 5 years, several key clinical trials of mRNA vaccines will be completed (especially trials for COVID-19).
More extensive clinical research will give us a more comprehensive understanding of mRNA vaccine platforms and various delivery systems. From improving the production capacity of mRNA and various drug-carrying materials, to screening potential carrier molecules and adjuvants; from improving the composition and construction of vaccines, to exploring the corresponding route of administration, and optimizing the core sequence encoding mRNA and verifying its delivery As for the mechanism of inducing immunity, the exploration and application of mRNA vaccines are far from mature enough, but its potential to become the vaccine of choice has been fully demonstrated.
mRNA vaccine: Mechanism Drug platform and Clinical prospects
(source:internet, reference only)
Disclaimer of medicaltrend.org