Influence of cell culture conditions on protein glycosylation
- Normal Liver Cells Found to Promote Cancer Metastasis to the Liver
- Nearly 80% Complete Remission: Breakthrough in ADC Anti-Tumor Treatment
- Vaccination Against Common Diseases May Prevent Dementia!
- New Alzheimer’s Disease (AD) Diagnosis and Staging Criteria
- Breakthrough in Alzheimer’s Disease: New Nasal Spray Halts Cognitive Decline by Targeting Toxic Protein
- Can the Tap Water at the Paris Olympics be Drunk Directly?
Influence of cell culture conditions on protein glycosylation
Influence of cell culture conditions on protein glycosylation. This article mainly discusses the influence of cell culture conditions on the quality of biological products, and focuses on the analysis techniques used for glycosylation and its accurate assessment.
Glycosylation may affect the half-life, immunogenicity, binding activity and stability of the protein. Protein glycosylation is a complex process, including the linking of carbohydrate moieties, and possibly via asparagine (N-linked) or serine/threonine (O-linked) amino acids in the protein structure.
In the process of mammalian cell culture, the use of different cell lines to culture may produce significant differences in the types of glycosylation that may occur. These differences in glycosylation may have a significant impact on the quality of the therapeutic protein produced. The choice of cell clones, the basal medium and feed medium used will also affect glycosylation.
The choice of host cells and bioreactor conditions used in the production of protein drugs will significantly affect the quality of protein products. This is not only determined by the complexity of the structure of the protein itself, but also by the specific post-translational modifications that may occur during cell culture. Among them, glycosylation is particularly important for the quality of the drug.
The influence of protein and cell culture on protein quality
Biopharmaceuticals are proteins with a polymer structure. The structure starts from the amino acid sequence (called the primary structure) by folding the amino acid chain into partial, secondary and tertiary conformations. Multichain proteins, such as IgG antibodies, also have a quaternary structure resulting from the structure between subunits.
The choice of host cell line for recombinant protein production firstly depends on the molecular properties of the protein. Certain bacteria can be used to produce simple proteins, that is, proteins consisting only of amino acid polymers, without post-translational modification (PTM) such as glycosylation, because most bacterial bacteria cannot perform glycosylation. The simple medium can be used for rapid production; however, the purification process can be challenging.
Using yeast can quickly produce proteins with original glycosylation. Usually together with baculovirus vectors in a transient manner, insect cells are mainly used for research and development and basic products. Mammalian cells are used to produce complex proteins, such as antibodies and enzymes, requiring complete PTM, including some complex carbohydrates.
Figure 1 below shows the structure, glycosylation sites and potential variability of IgG antibodies. Compared with small molecule drugs, proteins are fragile molecules and face multiple stability challenges. A typical glycoprotein (such as an IgG antibody) has many variable sites in its structure, including four chains, with a total molecular weight of 150,000 Da. In addition, several post-translational modifications of the protein chain may occur, such as oxidation and deamidation of specific amino acids. Each heavy chain can also have glycosylation sites.
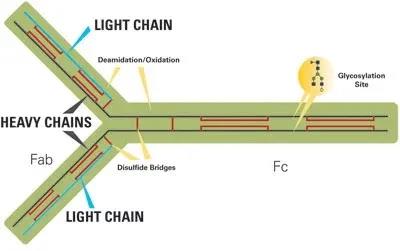
Why is glycosylation important?
Many complex proteins, such as antibodies and enzymes, are glycoproteins containing 2-30% carbohydrates. Glycosylation is a complex process in which carbohydrates are attached to proteins via asparagine (N-linked) or serine/threonine (O-linked) amino acids. Proteins may contain multiple types of sugars, each of which has multiple attachment sites. Variations in mammalian cells used in production can lead to subtle differences in glycosylation.
The choice of cell clone will affect the quality of the product. The glycosylation and PTM capabilities of each clone are slightly different, and their viability is different, resulting in differences in the intracellular degrading enzymes released into the medium. Therefore, it may be necessary to select the cell line not the cell clone with the highest expression, but the cell line that can achieve the desired protein quality.
Influence of cell culture conditions
Cell culture parameters that affect the type and degree of glycosylation include pH, CO2 content, dissolved oxygen (dO2), temperature, nutrient levels and types, the presence and types of glycan precursors, cell viability, as dying cells release degradation Enzyme, and process control level.
Compared with shake flasks, bioreactors can provide greater control over pH and dissolved gases, and therefore can achieve better process control, but shake flasks are more economical and can easily be used in larger quantities or larger scales.
Therefore, shake flasks are often used for early screening studies of media and feed conditions. By cooperating with the bioreactor, the growth and productivity can be improved by selecting the basal medium and the culture feed and the time of adding these feeds, so as to achieve the best cell culture. It can also optimize the oxygenation conditions of the bioreactor, such as CO2 level, pH, stirring, temperature, seeding density, and distribution ratio; use temperature changes to extend the viability of the cell culture during the production phase; and minimize the accumulation of growth-inhibiting metabolites such as ammonia.
Recently, the availability of micro-bioreactors provides an effective way to conduct multi-parameter studies. Therefore, a “design of experiment” (DoE) research method can be carried out, in which multiple interacting bioreactor conditions can be evaluated simultaneously. Such research requires the use of automated large numbers of bioreactors to make the process feasible.
Precautions for cell culture of biosimilars
The research and development of biosimilars can use a “generic” version of protein drugs, which must be highly similar to the original drug. The regulatory guidelines require extensive analysis and testing of the original drug, including a complete glycosylation profile.
The similarity with the original drug is crucial. This must start with clone selection and run through the entire development process. Therefore, instead of choosing the clone with the highest titer (as it is usually the case for innovative drugs), it is the first choice of biological similarity, which may mean that some high-expressing clones are not selected.
Glycosylation analysis technology
N-glycosylation occurs when carbohydrates are linked to asparagine with the consensus sequence asparagine-X-serine/threonine, where X is any amino acid other than proline. When the expressed protein is synthesized in the endoplasmic reticulum of the cell, the overall transfer of the pre-formed lipid-fixed conservative glycans occurs.
When the synthesized protein enters through the Golgi apparatus, the conserved N-linked glyan is “processed” by enzymes (glycosidase and glycosyltransferase). The existence of these processing enzymes, their relative levels and the accessibility of glycosylation sites on the protein to these enzymes determine the final glycosylation type of the protein.
O-glycosylation occurs on the amino acids serine and threonine, but does not conform to the linear consensus sequence. There are some rules that control this process. For example, there are often proline amino acids nearby in the glycosylation region, and tandem repeats of serine and theanine are also common.
N-glycans
Analysis of N-glycan analysis is the most active area of research. The techniques applicable to N-glycosylation analysis are also applicable to O-glycosylation.
The most abundant N-glycan structures in mammals are complex types, in which many N-acetylglucosamine structures are attached to the molecule and extend together with galactose, fucose and sialic acid residues to form two to Four antennae structure. The exact structure reflects the nature of the enzymes present in the cell type used in the expression process, and the precise environment the cell is in.
The International Conference on Harmonization (ICH) Quality Guide Q6B describes the types of analysis that should be performed on these structures to understand the amount of different types of monosaccharides present, the antenna distribution characteristics and connectivity of glycans, and the monosaccharide content in each structure, and Glycan structures at different glycosylation sites on the protein backbone.
Detailed analysis process:
The analysis of glycosylation involves many physical and enzymatic steps to release the glycans and then separate them from the peptides and any O-glycopeptides present in the mixture. O-glycans can be chemically released from these O-glycopeptides and purified separately from the rest of the peptide chain.
Then the separated N-glycans and O-glycans are permethylated and derivatized so that they can be analyzed by matrix-assisted laser desorption ionization mass spectrometry (MALDI-MS).
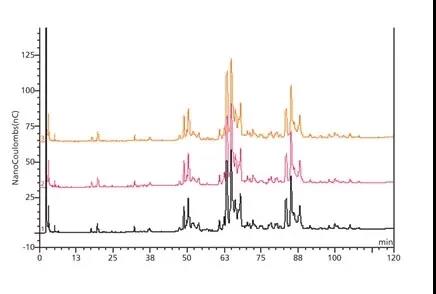
It is also possible to use chromatography to analyze samples instead of using permethylation methods. For example, high pH anion exchange chromatography with pulsed amperometric detection (HPAEC-PAD) can be used to analyze N-glycans. An example can be seen from the N-glycans released from bovine fetuin, as shown in Figure 2 below. This method provides consistent data in the three preparations, representing a series of cluster peaks of two, three, and four sialylated glycans (ie, two, three on the N-glycan in each cluster) And four sialic acid groups). The analysis gives some structural information based on the product produced, but little information on the exact nature of the glycan. Therefore, techniques like this are useful for comparison work, but do not provide much structural information to characterize molecules.
Figure 2 above shows the stacked high pH anion exchange chromatography and pulsed amperometric detection (HPAEC-PAD) chromatograms. It also shows the chromatograms of the glycans released in the three bovine fetuin preparations. In another method, the fluorescent label 2-aminobenzamide can be used to specifically label the released N and then these labeled N-glycans can be chromatographically separated.
The chromatographic eluent is then analyzed by mass spectrometry (MS) to identify the quality of individual structures. This is a useful technique because the chromatogram not only provides batch-to-batch comparisons, but also provides information about the quality of glycans in each peak.
The technology is very sensitive, so it is possible to resolve and identify two different forms of the so-called G1F structure, the monogalactosylated biantennary glycans found on antibodies. Both components have a galactose structure on either arm of the dual antenna structure, which can separate them. However, mass spectrometry must be further used to fully characterize the composition of these glycans.
Characterization using mass spectrometry
The aforementioned hypermethylation method allows the separation of glycans of similar quality in a non-derivatized state. For example, two glycans-have the same structure, except that one contains one N-acetylneuraminic acid (sialic acid) residue and the other contains two fucose residues-in their natural state, the molecular weight differs by only 1 Da, But when the difference is 13 Da, they have the same structure derivatization.
Matrix-assisted laser desorption ionization mass spectrometry (MALDI-MS) analysis can give an overall overview of the glycans present. Using the peak (mass) of spectral analysis, combined with the understanding of N-glycans, core structure and existing biosynthetic pathways, the composition of glycan monosaccharides can be derived.
Next, electrospray mass spectrometry (ES-MS) can be used to cleave the methylated glycans. This splitting effect is useful because the fracture path is clearly defined and well understood, depending on where the fracture occurs to produce a specific mass. The process depends on the exact nature of the N-glycan structure, so the quality of these fragments allows the antenna structure present on the glycan to be determined.
A third method, gas chromatography-mass spectrometry (GC-MS) can also be used to determine how monosaccharides are connected to each other within the glycan structure. These structural changes will have a great impact on the efficacy and properties of the drug.
By chemically modifying methylated glycans and attaching a specific “reporter group” where each monosaccharide is connected to each other, derivatives can be prepared, which can provide critical structural information when analyzed by mass spectrometry. Using an electron impact mass spectrometer, the fragmentation map showed characteristic fingerprints of different bonds. In addition, the GC device on the front end of the mass spectrometer can identify each of the different link structures with different reporter groups because they elute from the GC column at different times. The combination of GC retention time and mass spectrometric fingerprint fragmentation patterns can identify different monosaccharide bonds in the glycan population.
The final step of characterization is to determine the stereospecificity of any linkages in the structure, where the data indicate that potentially immunogenic epitopes may exist, such as GalαGal (a known immunogenic epitope). Treatment of glycans with specific exoglycosidase and analysis by MS before and after exposure can confirm the presence of α-linked galactose by showing mass transfer after incubation.
Conclusion
Compared with small molecule drugs, protein is inherently complex and its size is much larger, and most PTMs, including glycosylation, are characteristic of many protein therapies. Their appearance depends on the molecular properties of the protein. Selection of cell types and individual clones; and cell culture and bioreactor conditions. Extensive analytical assays are required to characterize protein therapeutics. Biosimilars require more analytical tests than innovative drugs because they need to prove the biological similarity to a given innovative molecule.
Mass spectrometry can provide structural information about the composition, structure, and bonds present in the N-glycan molecule, and the use of chromatography can provide a unique glycan profile due to the precise structure and associated interactions of the glycan. This profile can be used as a reference and can be compared with other batches. In addition, the use of LC/ES-MS for proteolytic digestion can isolate and identify glycosylation sites. A variety of techniques can then be used to analyze these sites to determine the nature of the glycans at each site and the specific population of glycans on the biopharmaceutical molecule.
Article Source:
1. D. Ghaderi et al., Biotech Gen. Eng. Rev. 28, pp. 147–176 (2012).
2. S.D. Jones et al., Am. Pharma. Rev. 18 (1), pp. 44–48 (2015).
3. FDA, Guidance for Industry, Scientific Considerations in Demonstrating Biosimilarity to a Reference Product (Rockville, MD, Apr. 2015).
4. FDA, Guidance for Industry, Quality Considerations in Demonstrating Biosimilarity of a Therapeutic Protein to a Reference Product (Rockville, MD, Apr. 2015).
(source:internet, reference only)
Disclaimer of medicaltrend.org